Unlocking the World of Physics Lab Instruments: A Comprehensive Guide
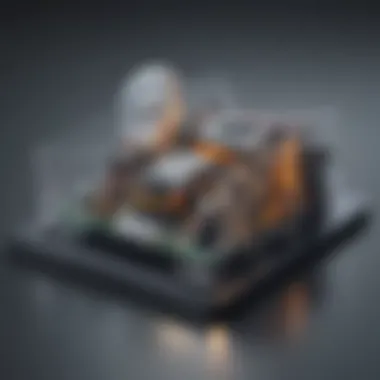
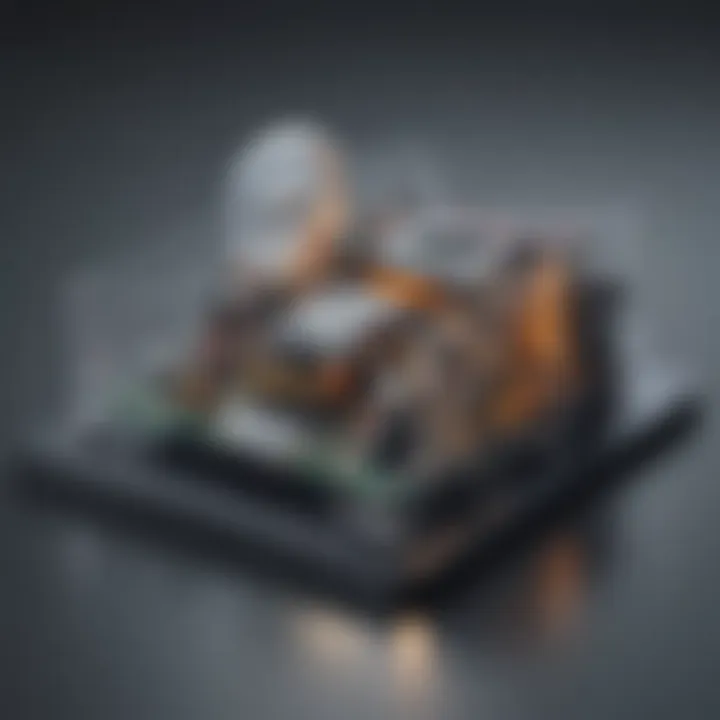
Overview of Topic
The realm of physics lab instruments is a vast and intriguing domain that forms the backbone 🔬 of experimental physics research. These instruments are vital tools utilized in analyzing and comprehending a wide array of physical phenomena. From the intricate workings of spectroscopes to the precise measurements obtained through oscilloscopes, each instrument contributes significantly to advancing our understanding of the physical world.
Fundamentals Explained
Exploring the core principles and theories that underpin physics lab instruments unveils a tapestry of intricate mechanisms guiding their functionality. Delving into key terminologies and definitions enables a grasp of the fundamental concepts essential for harnessing these tools effectively. Through mastering these basic concepts, individuals gain a foundational knowledge that aligns with the intricate workings of physics lab instruments, paving the way for subsequent exploration.
Practical Applications and Examples
Real-world case studies and applications highlight the indispensable nature of physics lab instruments in driving breakthroughs in scientific research. Hands-on projects and demonstrations offer a tactile understanding of how these instruments are employed in varying contexts. Accompanied by illustrative code snippets and implementation guidelines, these examples serve as stepping stones in navigating the practical terrain of utilizing physics lab instruments.
Advanced Topics and Latest Trends
Embracing cutting-edge developments within the realm of physics lab instruments unveils a trailblazing landscape. From advanced techniques to evolving methodologies, a glimpse into the latest trends propels individuals towards futuristic prospects. Intriguing insights into the future prospects and upcoming trends of these instruments shed light on the uncharted territories awaiting exploration.
Tips and Resources for Further Learning
For aficionados seeking to deepen their understanding and expertise in physics lab instruments, a curated list of recommended books, courses, and online resources stands as a beacon of knowledge. Equipped with tools and software tailored for practical usage within this domain, individuals are empowered to embark on a journey of continuous learning and exploration.
Physics lab instruments serve as crucial tools in experimental research, playing a fundamental role in unraveling intricate physical phenomena. Through the meticulous exploration of spectroscopes to oscilloscopes, researchers can conduct precise experiments, advancing the frontiers of physics.
Introduction to Physics Lab Instruments
Physics lab instruments are indispensable in experimental physics research, facilitating the study, analysis, and comprehension of various physical phenomena. The significance of these instruments lies in their ability to assist researchers in conducting accurate and insightful experiments, driving progress in the field of physics.
Understanding the Importance of Lab Instruments
Enhancing Experimental Precision
Enhancing experimental precision is a critical aspect of physics lab instruments as it ensures the accuracy and reliability of experimental results. By minimizing errors and uncertainties in measurements, enhanced precision allows researchers to draw valid conclusions and make significant contributions to the scientific community.
Facilitating Data Collection and Analysis
Facilitating data collection and analysis is another vital function of lab instruments. These tools streamline the process of gathering experimental data, making it easier for researchers to analyze and interpret the information effectively. By simplifying data handling, lab instruments enable researchers to extract valuable insights from their experiments.
Enabling Validation of Physical Theories
Lab instruments play a key role in validating physical theories by providing empirical evidence to support or refute scientific hypotheses. Through meticulous experimentation and data collection, these instruments help researchers verify the validity of existing theories, contributing to the advancement of physics knowledge.
Overview of Common Physics Lab Instruments
Spectrometer
Spectrometers are essential instruments in physics labs for analyzing the interaction of light with matter. By measuring the properties of light, such as wavelength and intensity, spectrometers provide valuable insights into the composition and structure of materials, making them indispensable for various experiments.
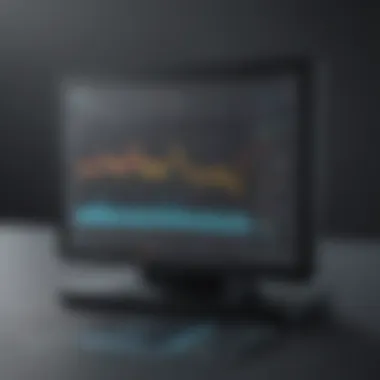
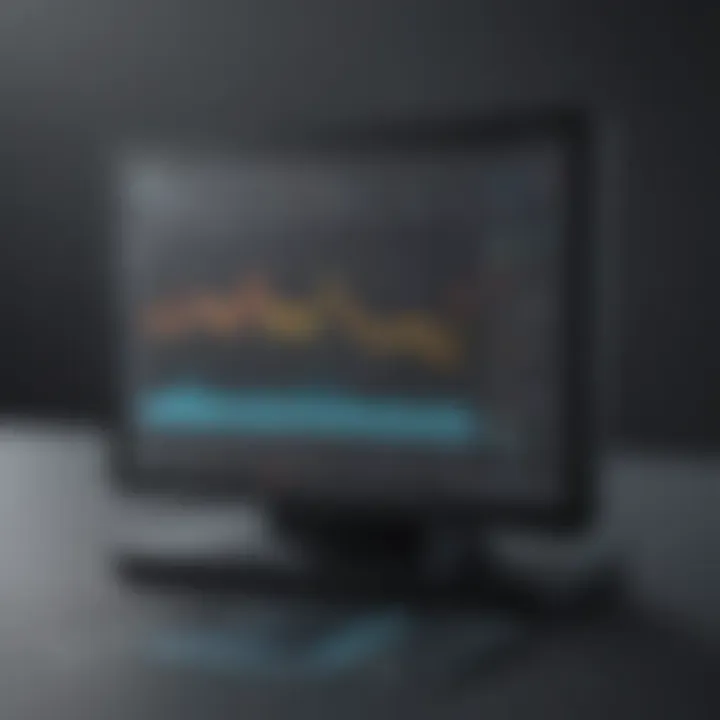
Microscope
Microscopes are indispensable tools for magnifying and observing small objects with enhanced resolution. In physics labs, microscopes play a crucial role in examining microscopic structures and phenomena, aiding researchers in visualizing and analyzing samples at the molecular and atomic levels.
Calorimeter
Calorimeters are specialized instruments used to measure heat transfer in chemical reactions. By quantifying the amount of heat released or absorbed during a reaction, calorimeters help researchers understand the thermodynamic properties of substances, enabling precise thermal analysis.
Oscilloscope
Oscilloscopes are vital tools for analyzing and visualizing electronic signals in physics experiments. By displaying signal waveforms in real-time, oscilloscopes allow researchers to study the behavior of electrical systems, making them essential for conducting accurate measurements and analyses.
Electron Microscope
Electron microscopes offer exceptional magnification and resolution for studying microscopic structures with high precision. By using electron beams to image samples at the nanoscale, electron microscopes provide detailed information about sample morphology and composition, enabling researchers to explore phenomena at the atomic level.
Types of Spectroscopes
In the realm of physics lab instruments, the study of different types of spectroscopes holds immense significance. Spectroscopes are crucial tools in experimental physics research as they allow scientists to analyze and understand various physical phenomena with enhanced precision. These instruments play a vital role in enhancing experimental accuracy, facilitating comprehensive data collection and analysis, and enabling validation of fundamental physical theories. Understanding the nuances of spectroscopes equips researchers with the tools necessary to conduct insightful experiments and contribute to the advancement of the field of physics.
Visible Spectroscopes
Prism Spectroscope
The Prism Spectroscope is a fundamental tool in the realm of spectroscopy, offering unique capabilities for analyzing visible light spectra. Its key characteristic lies in its ability to disperse light into its component colors, thus enabling researchers to study the spectral components of different samples accurately. This aspect makes the Prism Spectroscope a popular choice in physics lab experiments where the analysis of visible light spectra is crucial. One of the notable advantages of the Prism Spectroscope is its simplicity in design, making it easy to use for both beginners and experienced researchers. However, a limitation of the Prism Spectroscope is its inability to provide detailed spectral resolution compared to more advanced spectroscopic techniques.
Diffraction Grating Spectroscope
The Diffraction Grating Spectroscope is another essential instrument in spectroscopy that contributes significantly to the exploration of light spectra. What sets this spectroscope apart is its capability to diffract light using a series of closely spaced lines, allowing for precise spectral analysis and measurement. The key characteristic of the Diffraction Grating Spectroscope is its high spectral resolution, providing researchers with detailed information about the composition and properties of the light source under study. This aspect makes it a preferred choice in research settings where in-depth spectral analysis is required. Despite its exceptional resolving power, the Diffraction Grating Spectroscope may pose challenges in calibration and alignment, demanding careful handling and maintenance.
Infrared Spectroscopes
Fourier Transform Infrared Spectroscope
The Fourier Transform Infrared Spectroscope is a powerful tool in the study of infrared spectra, offering unique advantages in the analysis of molecular compositions and structures. Its key characteristic lies in its ability to rapidly acquire high-resolution spectra through Fourier transformation, enabling researchers to identify molecular fingerprints with precision. This feature makes the Fourier Transform Infrared Spectroscope a valuable asset in applications requiring detailed molecular analysis, such as organic chemistry and material science. However, one of the limitations of this spectroscope is its sensitivity to interference from atmospheric conditions, necessitating controlled environments for accurate measurements.
Near-Infrared Spectroscope
The Near-Infrared Spectroscope provides researchers with an invaluable tool for studying near-infrared light spectra and their applications in various scientific disciplines. Its key characteristic is the ability to analyze molecular vibrations and interactions in the near-infrared region, offering insights into material composition and structural properties. This feature makes the Near-Infrared Spectroscope a popular choice in fields such as pharmaceuticals, agriculture, and environmental science, where detailed analysis of organic compounds is essential. However, a drawback of this instrument is its limited resolution compared to other spectroscopic techniques, potentially affecting the accuracy of spectral measurements.
UV-Visible Spectroscopes
Single-beam Spectroscope
The Single-beam Spectroscope stands out as a versatile instrument for analyzing the ultraviolet and visible light spectra of various samples. Its key characteristic lies in its straightforward design, enabling researchers to quickly measure and analyze the absorption and emission spectra of different materials. This simplicity makes the Single-beam Spectroscope a preferred choice in educational settings and routine spectral analysis tasks. However, one of the limitations of this spectroscope is its potential susceptibility to background noise and signal interference, requiring careful adjustments during measurements.
Double-beam Spectroscope
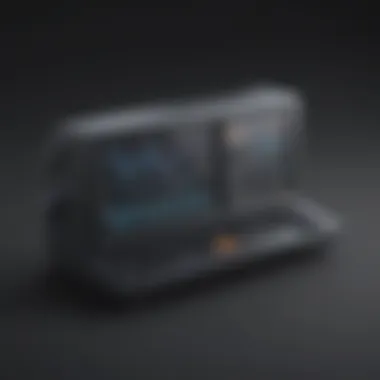
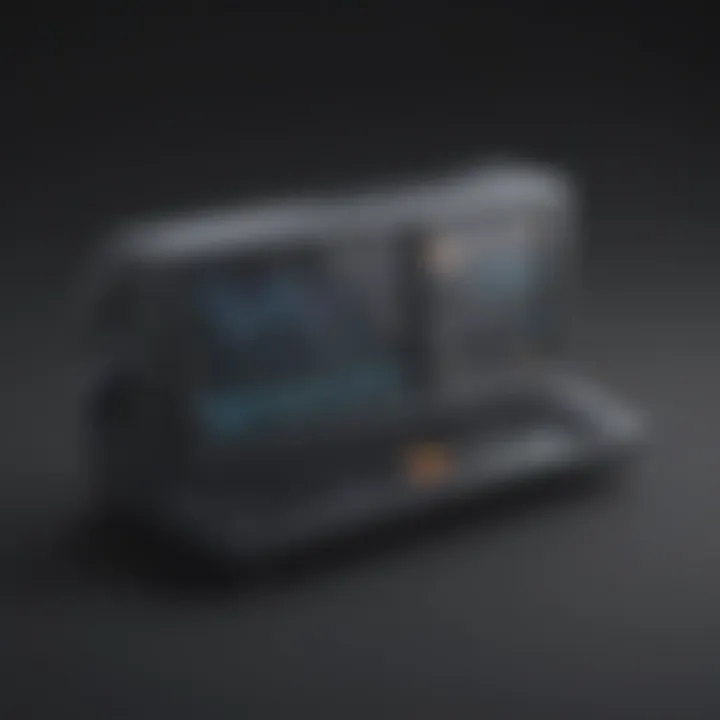
The Double-beam Spectroscope is a sophisticated instrument that offers enhanced capabilities for studying UV and visible light spectra with precision. Its key characteristic is the presence of two light beams – a sample beam and a reference beam – allowing for accurate comparison and measurement of spectral features. This dual-beam setup provides researchers with reliable data for quantitative analysis and spectral interpretation, making the Double-beam Spectroscope a valuable tool in analytical chemistry and material characterization. Despite its advanced features, the Double-beam Spectroscope may require regular calibration and maintenance to ensure consistent performance and measurement accuracy.
Functions of a Microscope
When delving into the realm of physics lab instruments, one cannot overlook the crucial role of microscopes. These essential tools play a significant part in magnifying and observing objects at a microscopic level. The functions of a microscope are instrumental in enabling researchers to visualize small-scale structures and analyze intricate details critical in various scientific experiments. By utilizing different types of microscopes, researchers can explore a wide range of samples, from biological specimens to materials, with exceptional clarity and precision.
Optical Microscopes
Optical microscopes, including compound microscopes, electron microscopes, and phase-contrast microscopes, offer remarkable capabilities in visualizing samples with exceptional detail. Each type of optical microscope brings unique features and advantages to the table, catering to different research needs and scientific applications. In this section, we will delve deeper into the specific aspects of each optical microscope type to understand their contributions to experimental physics research.
Compound Microscope
The compound microscope is a cornerstone instrument in scientific research, renowned for its ability to magnify objects with high resolution. Its key characteristic lies in its optical configuration, which includes multiple lenses that work in tandem to produce a magnified image. This configuration allows researchers to observe specimens with enhanced clarity and detail, making it an invaluable choice for studying biological samples and observing various microscopic structures within materials. Despite its powerful magnification capabilities, the compound microscope may have limitations in terms of depth of field and field of view, factors that researchers consider when selecting the appropriate microscope for their experiments.
Electron Microscope
The electron microscope stands as a revolutionary tool in microscopy, exploiting electron beams to achieve unprecedented magnification levels. Its key characteristic lies in its electron-optical system, which replaces traditional lenses with magnetic coils that manipulate electron beams. This unique feature enables the electron microscope to visualize samples at nanoscale resolutions, uncovering intricate details of subcellular structures and nanomaterials that are beyond the reach of optical microscopes. However, the electron microscope's high vacuum requirements and complex sample preparation procedures are aspects that researchers need to consider when utilizing this advanced imaging instrument.
Phase-Contrast Microscope
The phase-contrast microscope introduces a novel technique for viewing transparent samples without the need for staining or labeling. Its key characteristic lies in its specialized phase-contrast optics, which enhance the contrast of transparent specimens by exploiting phase shifts in light passing through them. This innovative feature makes the phase-contrast microscope ideal for observing live cells, microorganisms, and other translucent samples in their natural state. While offering non-invasive imaging capabilities, researchers should be mindful of potential phase artifacts that may affect the accuracy of their observations in certain experimental setups.
Scanning Probe Microscopes
Apart from optical microscopes, scanning probe microscopes, including atomic force microscopes and scanning tunneling microscopes, provide exceptional capabilities in imaging surfaces at the atomic and molecular levels. These cutting-edge instruments offer detailed insights into nanostructures and surface properties, paving the way for groundbreaking research in nanoscience and materials analysis. Let's explore the unique features and advantages of each scanning probe microscope type in the context of experimental physics research.
Atomic Force Microscope
The atomic force microscope revolutionizes surface imaging by utilizing a sharp probe to trace surface contours at atomic resolution. Its key characteristic lies in its sensitive cantilever-based detection system, which measures surface forces with exceptional accuracy. This capability enables researchers to investigate nanoscale interactions, map surface topographies, and study mechanical properties of materials at the atomic level. Despite its impressive imaging capabilities, the atomic force microscope may have limitations in imaging speed and complex data interpretation, factors researchers need to consider when designing experiments.
Scanning Tunneling Microscope
The scanning tunneling microscope offers powerful imaging capabilities by exploiting quantum tunneling phenomena to image conductive surfaces with atomic precision. Its key characteristic lies in its sharp metal tip that scans the surface while maintaining a constant tunneling current - a technique that allows researchers to visualize individual atoms and manipulate them on surfaces. This exceptional feature makes the scanning tunneling microscope indispensable for studying surface structures, molecular interactions, and nano-scale phenomena. However, the scanning tunneling microscope's reliance on conducting samples and its sensitivity to environmental conditions are aspects researchers should account for in their experimental setups.
Applications of a Calorimeter
Bomb Calorimeter
Measuring Heat Transfer in Chemical Reactions
The utilization of bomb calorimeters for measuring heat transfer in chemical reactions is a pivotal aspect of experimental research in thermodynamics. This method involves encapsulating the reactants within a sealed environment called a bomb, where the reaction takes place under controlled conditions. By carefully monitoring the temperature changes that occur during the reaction, scientists can accurately calculate the heat produced or absorbed. The bomb calorimeter's sealed design ensures minimal heat loss to the surroundings, allowing for precise measurements of heat transfer. This technique is particularly valuable for studying reactions with high precision, making it an indispensable tool for researchers investigating the energetics of chemical processes.
Differential Scanning Calorimeter
Studying Thermal Transitions
The use of a differential scanning calorimeter (DSC) is instrumental in studying thermal transitions in various materials, offering unique insights into their physical and chemical properties. DSC works by comparing the heat flow into a sample with that of a reference material, enabling the detection of phase transitions, crystallization processes, and thermal stability. This technique provides detailed information on the heat capacity and enthalpy changes associated with these transitions, allowing researchers to characterize the material's behavior under different temperature conditions. Differential scanning calorimetry is widely adopted in material science, pharmaceuticals, and polymer research due to its ability to accurately assess thermal properties and phase changes in a wide range of substances.
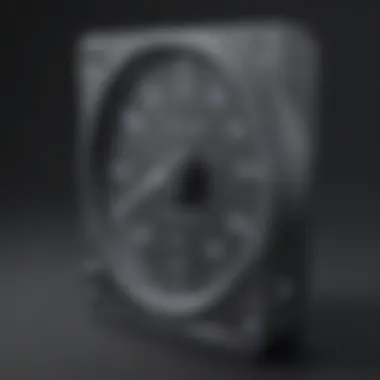
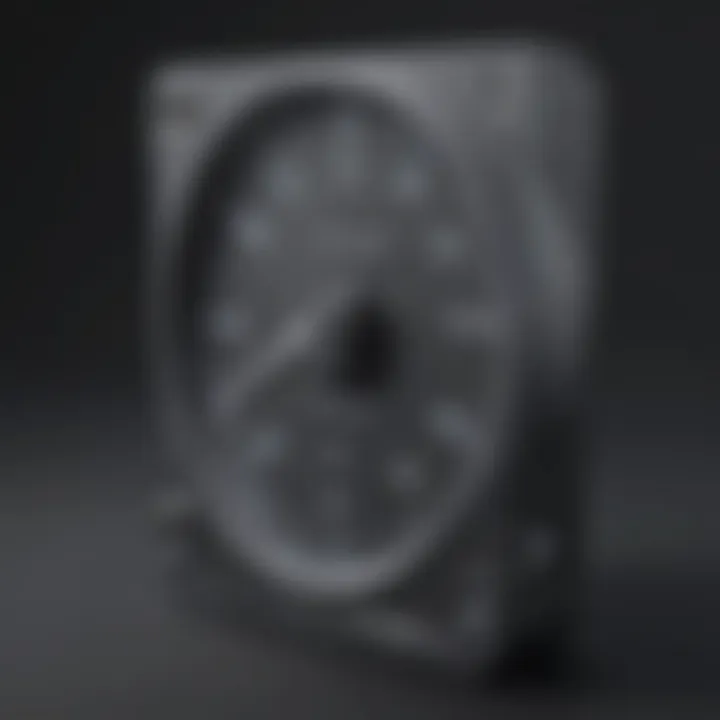
Weaving through the intricate details of calorimetry, researchers unlock a wealth of knowledge on heat transfer, enthalpy changes, and thermal behaviors, paving the way for groundbreaking discoveries in the field of experimental physics.
Oscilloscope: A Tool for Waveform Analysis
In the realm of physics lab instruments, the oscilloscope emerges as a pivotal tool for analyzing waveforms, making it a cornerstone of experimental research. Its significance lies in its ability to accurately measure and display voltage signals over time, providing researchers with crucial insights into the behavior of electric circuits and signals. By capturing and visualizing waveforms, oscilloscopes facilitate the interpretation of complex signals, essential for various physics experiments and circuit analysis.
Types of Oscilloscopes
Analog Oscilloscope
The analog oscilloscope stands out for its fundamental role in waveform analysis, known for its reliable representation of signal amplitude variations. Its key characteristic lies in its direct and real-time display of analog signals, making it a preferred choice for scenarios demanding continuous signal monitoring. The unique feature of analog oscilloscopes is their smooth display of waveform details, offering a straightforward interpretation of signal characteristics. While analog oscilloscopes excel in displaying rapid signal changes accurately, their main drawback lies in the limited ability to store and analyze waveform data for further scrutiny.
Digital Storage Oscilloscope
Conversely, the digital storage oscilloscope introduces a modern approach to waveform analysis by digitizing and storing input signals for precise analysis and detailed investigations. Its standout feature is the capacity to capture and store waveforms in digital format, enabling researchers to conduct in-depth signal analysis and measurements. Digital storage oscilloscopes are highly valued for their ability to save waveform data for future reference and post-analysis, enhancing research efficiency and data reproducibility. While digital storage oscilloscopes excel in capturing and analyzing complex waveforms with greater accuracy, they may face limitations in capturing high-speed signals due to their sampling rate constraints.
Key Features and Functions
Waveform Visualization
The aspect of waveform visualization plays a pivotal role in enabling researchers to interpret and analyze complex signal patterns effectively. Its key characteristic lies in providing visual representations of voltage signals over time, allowing for the identification of signal anomalies and irregularities. Waveform visualization is a valuable choice for this article due to its intuitive display of waveform details, supporting insightful waveform analysis. The unique feature of waveform visualization is its ability to enhance signal comprehension and aid researchers in making data-driven decisions. While waveform visualization excels in providing immediate insights into signal behavior, it may encounter challenges when dealing with high-frequency signals that require specialized equipment for accurate representation.
Frequency Measurement
Frequency measurement holds essence in determining the rate at which a signal oscillates, paving the way for precise signal characterization and frequency analysis. Its key characteristic lies in quantifying the number of signal cycles per unit time, aiding researchers in understanding signal periodicity and frequency modulation. Frequency measurement serves as a beneficial choice for this article by enabling researchers to quantify signal frequencies accurately and evaluate signal stability over time. The unique feature of frequency measurement is its capability to identify signal harmonics and frequency components, essential for advanced signal processing and modulation analysis. While frequency measurement excels in offering precise frequency quantification, it may face challenges when measuring high-frequency signals due to instrumentation limitations.
Signal Analysis
Signal analysis emerges as a sophisticated process that involves the examination and interpretation of signal properties for insightful decision-making and signal optimization. Its key characteristic lies in dissecting signal parameters such as amplitude, frequency, and phase, allowing researchers to extract valuable signal information and detect abnormalities. Signal analysis proves to be a popular choice for this article due to its indispensable role in signal processing and quality assessment. The unique feature of signal analysis is its ability to uncover hidden signal patterns and aberrations, facilitating in-depth signal diagnostics and troubleshooting. While signal analysis excels in unveiling signal intricacies, it may encounter complexities when dealing with noisy or distorted signals that require advanced algorithms for accurate analysis.
Exploring Electron Microscopes
In the vast landscape of physics lab instruments, electron microscopes stand out as powerful tools that have revolutionized scientific explorations. The section on exploring electron microscopes delves into the significance of these instruments within the context of this comprehensive article, shedding light on their specific elements, benefits, and considerations. Through a detailed analysis of transmission electron microscopes (TEM) and scanning electron microscopes (SEM), readers will gain a profound understanding of how these instruments contribute to advancements in experimental physics research.
Transmission Electron Microscope (TEM)
High-Resolution Imaging
High-resolution imaging in transmission electron microscopes (TEM) plays a pivotal role in enabling researchers to observe the intricacies of specimens at a molecular and even atomic level. The key characteristic of high-resolution imaging lies in its ability to visualize ultrafine details with remarkable clarity, allowing for deeper insights into the composition and structure of materials. This feature is particularly beneficial in this article as it provides a comprehensive view of the intricate world of nanoscale physics, facilitating a thorough exploration of various physical phenomena. Despite its advantages in delivering precise imaging, high-resolution techniques may pose challenges in handling specimen sensitivity and imaging at a rapid pace.
Analytical Capabilities
When it comes to analytical capabilities, transmission electron microscopes (TEM) offer a treasure trove of functionalities that aid in characterizing materials with unparalleled accuracy. The key characteristic of these capabilities lies in their capacity to perform elemental analysis, diffraction studies, and compositional mapping at nanoscale dimensions, providing researchers with invaluable data for scientific investigations. In the context of this article, the analytical prowess of TEM serves as a vital tool for elucidating complex physical theories and validating experimental results. Despite its numerous benefits, these analytical capabilities may present challenges in terms of sample preparation and data interpretation.
Scanning Electron Microscope (SEM)
Surface Imaging
Surface imaging, a fundamental aspect of scanning electron microscopes (SEM), allows researchers to visualize the topography of specimens with exceptional clarity and depth. The key characteristic of surface imaging lies in its ability to generate detailed three-dimensional images of sample surfaces, enabling a comprehensive understanding of surface structures and features. This feature proves invaluable within this article as it facilitates the exploration of surface phenomena and interfaces in various materials, offering insights into surface properties and behaviors. While surface imaging enhances the visualization of samples, it may encounter limitations in terms of resolution and imaging depth.
Elemental Analysis
The elemental analysis capability of scanning electron microscopes (SEM) empowers researchers to identify and quantify the elemental composition of materials with precision. The key characteristic of elemental analysis lies in its ability to perform energy-dispersive X-ray spectroscopy (EDS) and wavelength-dispersive X-ray spectroscopy (WDS) to determine elemental distributions within samples. In the context of this article, elemental analysis plays a crucial role in elucidating the chemical composition and structure of materials under study, aiding in the comprehensive analysis of physical properties. Despite its advantages in determining elemental composition, elemental analysis may face challenges related to spectral interferences and data interpretation.