The Structure of the Atom: A Comprehensive Exploration
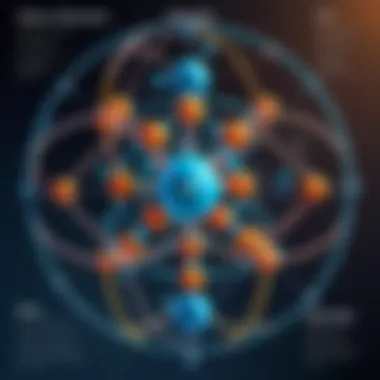
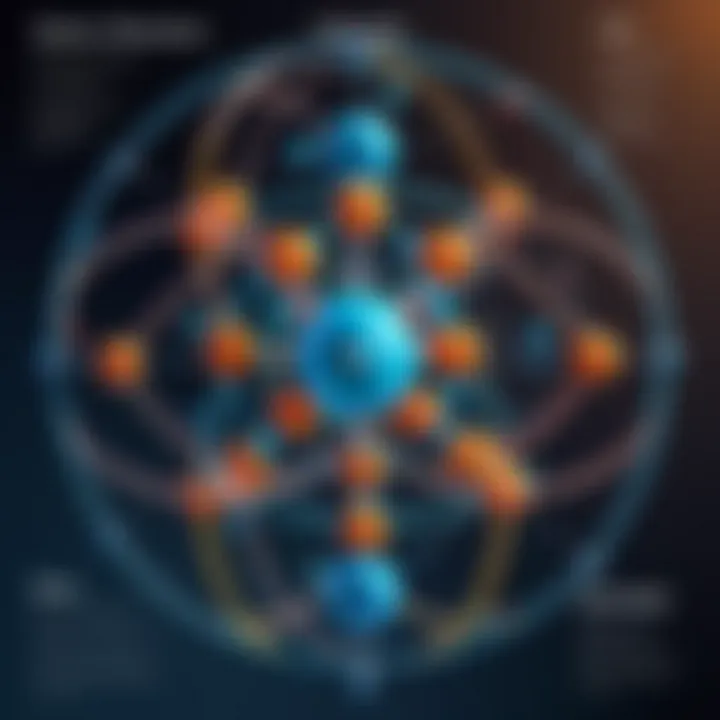
Overview of Topic
Atoms form the very foundation of matter. Understanding their structure is essential not only for students of science but also for professionals across many disciplines. This article explores the complexities of atomic structure, emphasizing its relevance to technology and modern applications.
The significance of atomic theory extends into various fields including physics, chemistry, and engineering. In an age where advancements in technology demand a deeper grasp of fundamental concepts, the study of atoms serves as a crucial pillar. The journey into atomic understanding began centuries ago, evolving from early philosophical notions to sophisticated quantum theories. Understanding this evolution aids in appreciating the current frameworks of atomic research.
Fundamentals Explained
At its core, an atom consists of three primary subatomic particles: protons, neutrons, and electrons. Protons are positively charged particles located in the nucleus, while neutrons are neutral particles also found within the nucleus. Electrons carry a negative charge and orbit the nucleus, forming an atomic cloud.
The atomic number determines the identity of an element, defined by the number of protons in the nucleus. An element's isotopes, which vary in neutron count, contribute to its stability and are vital in applications like nuclear energy and medical imaging.
Key Terminology
- Protons: Positively charged particles in the nucleus.
- Neutrons: Neutral particles in the nucleus giving mass.
- Electrons: Negatively charged particles orbiting the nucleus.
- Isotopes: Variants of elements with different neutron counts.
Practical Applications and Examples
Understanding atomic structure allows for applications in numerous fields. For instance, in nuclear energy, isotopes are manipulated to release energy efficiently. Medical imaging techniques like PET scans employ isotopes to trace biological processes.
In electronics, the arrangement of electrons plays a crucial role in conductivity and insulation properties. A clear example can be seen in semiconductor materials, which rely on controlled atomic structuring for performance in devices like transistors and diodes.
Advanced Topics and Latest Trends
The field of atomic research is not stagnant. Recent advancements include explorations into quantum computing, where understanding electron behavior can revolutionize processing power. Similarly, research into quantum entanglement opens pathways for breakthroughs in communication technologies.
Emerging methodologies in atomic interactions are vital for developing new materials, particularly in nanotechnology. Using atomic-level manipulation, scientists create compounds with unique properties for various technological applications.
Tips and Resources for Further Learning
To deepen your understanding, consider exploring the following resources:
- Books: "Atomic Structure and the Periodic Table" provides foundational knowledge in an easily digestible format.
- Courses: Online platforms like Coursera or edX offer courses on atomic theory.
- Tools: Simulation software such as PhET Interactive Simulations can aid in visualizing atomic structures.
Additional resources include:
Understanding atomic structure is essential not only for academic pursuits but also for practical innovations across modern technology.
Prelims to Atomic Structure
Understanding atomic structure is essential in the field of science. Atoms are the building blocks of matter, comprising all substances around us. This section aims to introduce the basic concept of atoms and their significance within various scientific disciplines. The structure of the atom serves as a fundamental principle influencing chemistry, physics, biology, and engineering.
Definition of an Atom
An atom is the smallest unit of an element that retains its chemical properties. It consists of a nucleus, which contains protons and neutrons, surrounded by electrons that occupy regions known as orbitals. The atom is often described as the basic unit of elements. Each element in the periodic table is defined based on its atomic structure, particularly the number of protons present in its nucleus. This number, known as the atomic number, fundamentally determines the identity of an element.
Significance in Science
The importance of studying atomic structure transcends mere definitions. It provides a foundation for understanding numerous scientific phenomena. The arrangement of atoms affects how substances interact with each other and dictates reactions in chemistry. Knowledge of atomic structure is crucial for advancements in fields such as quantum mechanics, which offer insights into behavior at subatomic levels.
Key reasons why this topic is significant include:
- Foundation of Chemistry: Understanding atoms is vital for grasping chemical reactions and bonding.
- Technological Development: Insights into atomic structure spur innovations in nanotechnology and materials science.
- Medical Applications: Knowledge of atomic behavior is applied in medical imaging and radiation therapy.
"The study of atoms is fundamental to the development of scientific knowledge that drives technological innovation and improvement in quality of life."
With this overview, we can delve deeper into the components that make up the atom, enhancing our comprehension of their roles and implications in science and beyond.
Components of the Atom
The examination of atomic structure fundamentally revolves around understanding its components. This understanding is critical for appreciating how atoms interact and form the essential building blocks of matter. Each component plays a distinct role, contributing to the atom's identity and behavior. In this section, we delve into the crucial elements that make up an atom: protons, neutrons, and electrons. Analyzing these subatomic particles provides insights into their properties and the overall significance of atomic structure in various scientific and practical contexts.
Protons
Charge and Mass
Protons are positively charged particles found in the nucleus of an atom. Their charge is a defining characteristic. It influences the electromagnetic interactions that occur during atomic bonding. Protons have a mass approximately 1836 times greater than that of electrons. This significant mass contributes to the overall mass of the atom, as they reside in the dense nucleus. The presence of protons is crucial; they define the atomic number and, thus, the identity of the element. Without them, we would not have clear identity among elements, making them a central focus of atomic structure.
Location in the Atom
The location of protons within the nucleus is vital for several reasons. The nucleus acts as the dense core of the atom, containing the majority of its mass. This centrality directly influences atomic stability. The location allows for interactions with both neutrons and electrons. It also determines how protons affect the arrangement of electrons around the nucleus, shaping the atom’s chemical properties. The positioning of protons contributes fundamentally to the theories of atomic structure, making their location an essential part of the narrative.
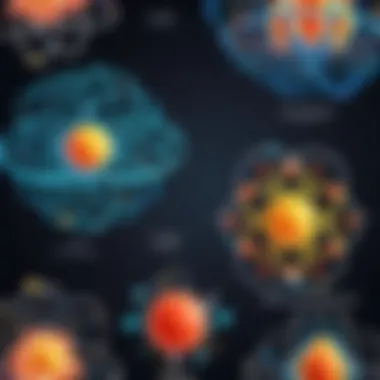
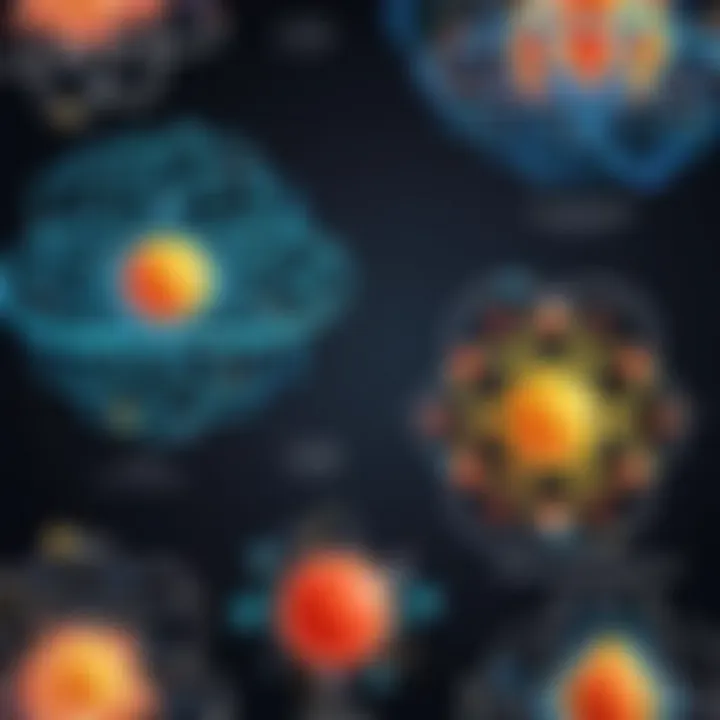
Role in Element Identity
Protons are fundamentally linked to the identity of an element. The number of protons in an atom determines its chemical properties and its behavior in reactions. This characteristic makes protons extremely important in the study of chemistry and physics. An atom's identity can change dramatically if the number of protons changes, thereby transforming it into a different element. This role shapes many scientific disciplines, showing that protons are more than just components; they are the essence of identity within atomic structure.
Neutrons
Neutral Charge
Neutrons carry no electric charge. This neutrality is a critical feature, allowing neutrons to exist in close proximity to positively charged protons without repelling them. Neutrons significantly enhance the stability of an atomic nucleus. They absorb the repulsive forces among protons, allowing the nucleus to maintain integrity. This unique charge property makes neutrons a vital element in understanding atomic interactions and stability.
Importance for Atomic Stability
The presence of neutrons is crucial for the stability of the nucleus. Without them, protons would repel each other due to their like charges. Neutrons help balance these forces, thus contributing to a stable atomic structure. Variations in the number of neutrons lead to isotopes, which can have diverse properties. Therefore, understanding this importance offers insights into nuclear reactions and stability, which are pivotal in fields such as nuclear physics and chemistry.
Variations and Isotopes
Isotopes are variations of an element that differ in the number of neutrons. This variation affects their mass and stability. Some isotopes are stable, while others are radioactive, decaying over time. Isotopes find numerous applications in medicine, such as in radiometric dating or cancer treatment. Understanding isotopes enhances knowledge about elements and their behaviors, presenting practical implications in different scientific fields.
Electrons
Negative Charge
Electrons are negatively charged particles orbiting the nucleus. Their charge is fundamental in the process of atomic interactions and bonding. The negative charge allows electrons to be attracted to protons in the nucleus, defining the atom’s structure. This interaction plays an essential role in forming bonds with other atoms, making electrons central to understanding chemical reactions and atomic behavior.
Electron Cloud Concept
Electrons do not occupy fixed orbits; instead, they exist in a cloud-like region around the nucleus. This model, known as the electron cloud model, illustrates the probability distribution of where an electron may be found. This concept is beneficial as it provides a more accurate understanding of electron behavior. This perspective is essential in fields such as chemistry and materials science, as it influences atomic bonding and molecular dynamics.
Energy Levels
Electrons reside in specific energy levels or shells around the nucleus. Each energy level has a distinct energy associated with it, which dictates the electron's behavior. Electrons can move between levels by absorbing or releasing energy. Understanding these energy levels is vital for explaining chemical reactivity, as it determines how atoms interact with one another. Such insights are significant in various scientific and technological applications, including semiconductor design and biochemical processes.
Historic Atomic Models
The evolution of atomic theory is significant in understanding the structure of the atom. Each model represents a leap in scientific thought and enhances our knowledge of atomic behavior. The historic atomic models laid the groundwork for modern atomic physics. They spotlight how human inquiry into the nature of matter has shaped scientific principles. Here, we delve into four primary atomic models that have contributed to our current understanding: Dalton's model, Thomson's plum pudding model, Rutherford's nuclear model, and the Bohr model.
Dalton's Model
John Dalton proposed his atomic model in the early 19th century. His work was crucial as it introduced the idea that matter is made up of atoms. Dalton's model depicted atoms as solid, indivisible spheres. This model supported the notion of conservation of mass and introduced atomic weights for different elements.
An important aspect of Dalton's model is the concept of the atomic theory, which states:
- Each element consists of identical atoms.
- Atoms of different elements are distinct from one another.
- Atoms combine in fixed ratios to form compounds.
While Dalton's vision laid the foundation, it lacked nuances regarding atomic structure, such as the internal arrangement of protons, neutrons, and electrons.
Thomson's Plum Pudding Model
In 1897, J.J. Thomson discovered the electron, fundamentally altering atomic theory. He proposed the plum pudding model in 1904. This model suggested that an atom is a sphere of positive charge with electrons embedded within, similar to plums in a pudding. This idea presented an early attempt to describe internal atomic structure, shifting the focus from indivisible atoms to subatomic particles.
The significance of this model includes:
- Introduction of electrons as fundamental components of atoms.
- Highlighting the existence of positive charge in atoms.
Despite its innovative approach, the plum pudding model was eventually challenged because it could not adequately explain the results of further experiments, particularly those conducted by Rutherford.
Rutherford's Nuclear Model
Ernest Rutherford conducted his famous gold foil experiment in 1909. The results invalidated the plum pudding model, leading to his nuclear model. Rutherford revealed that most of an atom's mass is concentrated in a small, dense nucleus surrounded by electrons. This nucleus contains protons and neutrons, which was a significant expansion of our understanding of atomic structure.
Key takeaways from Rutherford's model:
- Atoms consist of a tiny, positively charged nucleus.
- Electrons orbit the nucleus at a distance.
This model formed the basis for modern atomic structure, emphasizing the nucleus's vital role in atomic behavior. It set the stage for more detailed studies of atomic structure.
Bohr Model
Niels Bohr improved upon Rutherford's nuclear model in 1913 by introducing quantized energy levels for electrons. In his model, electrons orbit the nucleus in fixed paths, or shells, and can jump between levels by absorbing or emitting energy. This assumption resolved issues related to atomic stability and emission spectra.
Features of Bohr's model include:
- Electrons occupy specific energy levels, leading to stable atom behavior.
- Energy absorption or release causes transitions between levels.
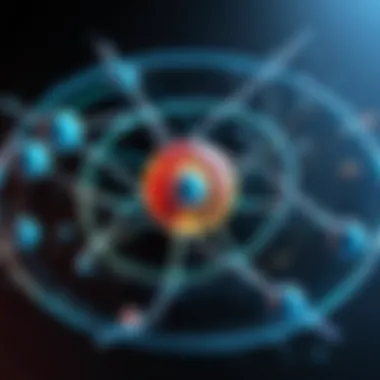
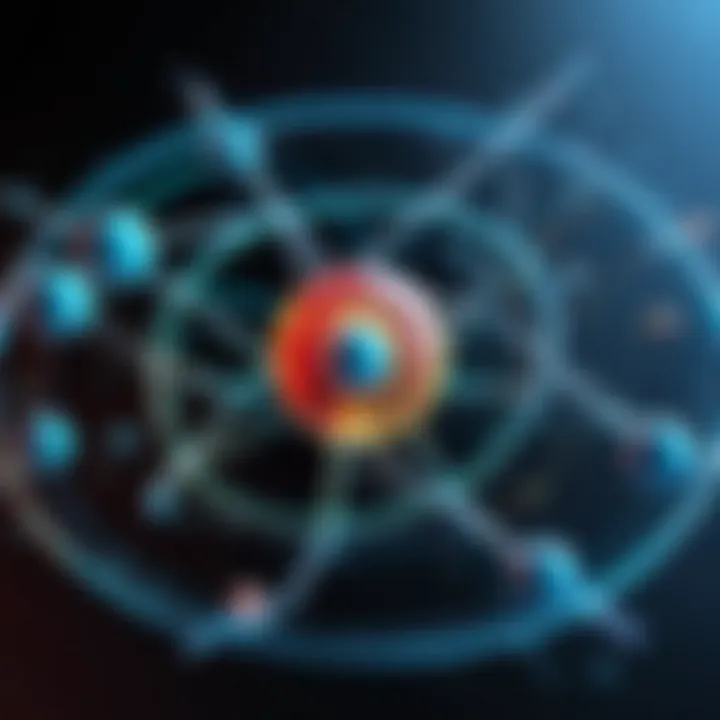
Although Bohr's model proved effective for hydrogen and several other elements, it has limitations when applied to more complex atoms. Nevertheless, it remained influential in the development of quantum mechanics and further atomic theories.
The historic atomic models showcase how scientific thought evolves. Each model contributes to our understanding of atomic structure, from indivisible atoms to complex quantum systems.
Understanding these models is essential for grasping more advanced concepts in atomic structure and quantum mechanics. They emphasize the journey of scientific discovery and the ongoing quest for knowledge.
Quantum Mechanics and Atomic Structure
Quantum mechanics plays a crucial role in understanding atomic structure. It shifts the perspective from classical physics to a more nuanced view of how atoms behave. In this framework, the atom is no longer depicted simply as a mini-solar system with electrons orbiting a nucleus; rather, it introduces principles that describe behaviors and interactions at the subatomic level. Such understanding is not only fundamental in physics but also impacts fields like chemistry, material science, and even modern computing.
Wave-Particle Duality
The concept of wave-particle duality is one of the cornerstone principles of quantum mechanics. It posits that every particle or quantum entity can be described as either a particle or a wave. This duality is vividly illustrated in experiments such as the double-slit experiment, which shows that light and matter can exhibit characteristics of both waves and particles depending on the observation setup. In the context of atomic structure, this means that electrons do not follow fixed paths around the nucleus, as one might picture planets orbiting a sun.
Instead, they exist in a state described by a probability wave, where the likelihood of locating an electron in a specific region around the nucleus can be calculated, creating what we refer to as the electron cloud. Understanding this concept transforms how atomic interactions and bonding are perceived, impacting both theoretical physics and practical applications.
Heisenberg Uncertainty Principle
The Heisenberg Uncertainty Principle further underlines the challenges in measuring atomic properties. It states that the position and momentum of a particle cannot be simultaneously known with arbitrary precision. This intrinsic limitation implies that attempting to pinpoint an electron's exact position disturbs its momentum, and vice versa.
In atomic theory, this principle is significant because it offers insights into the behavior of electrons and how they interact with other particles. It emphasizes the probabilistic nature of quantum systems, challenging classical determinism. This principle reshapes our understanding of chemical reactions and atomic interactions, particularly in complex systems where multiple forces act simultaneously.
Quantum Orbitals
Quantum orbitals are mathematical functions that describe the likelihood of finding an electron in a particular region of space around the nucleus. Unlike fixed orbits in the Bohr model, these orbitals provide a more accurate picture of electron distributions.
Each orbital has a defined shape and energy level, differentiating among key types such as s, p, d, and f orbitals.
- s Orbitals: Spherical in shape, accommodating two electrons.
- p Orbitals: Dumbbell-shaped, consisting of three orientations and holding up to six electrons.
- d and f Orbitals: More complex shapes and higher energy states, which are crucial for understanding transition metals and their properties.
Understanding quantum orbitals is vital for predicting how atoms bond and interact, which is foundational in chemistry and materials science. They allow chemists to determine reactivity and bonding patterns, essential for both theoretical studies and practical applications in technology.
Atomic Number and Mass Number
Understanding the atomic number and mass number is essential in the field of atomic structure. These two numbers provide critical insights into the characteristics of an atom, such as its identity and stability. They help both in the classification of elements and in predicting how different elements interact in chemical reactions.
Definition of Atomic Number
The atomic number is defined as the number of protons found in the nucleus of an atom. It is often denoted by the symbol "Z." This number is unique for each element and determines the identity of the element; for example, hydrogen has an atomic number of 1, while helium has an atomic number of 2. This simple numerical value is crucial. It not only defines the element but also influences its chemical behavior and properties.
- The atomic number also dictates the number of electrons in a neutral atom. This balance of protons and electrons results in a neutral overall electrical charge, which is vital for the atom's stability.
- Understanding atomic numbers can aid in the classification of elements on the periodic table. Each element’s position correlates directly with its atomic number, establishing a systematic approach to studying chemical properties and relationships.
Definition of Mass Number
Mass number refers to the total number of protons and neutrons in the nucleus of an atom. It is represented by the symbol "A." Unlike atomic number, mass number can vary even among atoms of the same element, due to the presence of isotopes. For instance, carbon has a mass number of 12, consisting of 6 protons and 6 neutrons; however, it can exist in a form known as carbon-14, which has 6 protons and 8 neutrons.
- Mass number is important for various calculations and applications. It plays a critical role in understanding atomic stability and radioactive decay, especially when evaluating isotopes that may emit radiation or undergo nuclear reactions.
- Knowing the mass number allows chemists and physicists to conduct more precise calculations and understand the processes that underlie chemical reactions and bonding.
"The distinction between atomic number and mass number is fundamental to understanding the behavior of elements and compounds in nature."
In essence, both atomic number and mass number are gateways to deeper insights into atomic structure and its significance in scientific exploration. Their definitions, implications, and uses reveal the complexity and elegance of atomic theory, serving as foundational concepts that inform our understanding of matter.
Isotopes and Their Significance
Isotopes are fundamentally related to the atomic structure and play a crucial role in various scientific and practical applications. Understanding isotopes begins with recognizing that they are variants of a particular chemical element. Isotopes share the same number of protons but differ in the number of neutrons, resulting in a variation in atomic mass. This subtle difference can have profound implications in fields such as medicine, environmental science, and nuclear energy.
In this section, we explore the definition of isotopes, provide examples, and examine their applications across different industries.
Definition and Examples
An isotope can be defined as an atom that has the same number of protons as another atom of the same element but a different number of neutrons. For instance, Carbon-12 and Carbon-14 are both isotopes of carbon. They each contain six protons; however, Carbon-12 has six neutrons while Carbon-14 has eight. This variance is what distinguishes their atomic masses and leads to different nuclear properties.
Isotopes can be classified as stable or unstable. Stable isotopes do not undergo radioactive decay, while unstable ones do, resulting in the emission of radiation. Common examples of stable isotopes include Carbon-12 and Oxygen-16, whereas some unstable isotopes include Uranium-235 and Carbon-14.
Applications in Medicine and Industry
Isotopes have vast applications, particularly in the fields of medicine and industry, reflecting their importance in modern society.
- Medical Imaging: One prominent use of isotopes is in medical imaging techniques, such as Positron Emission Tomography (PET) scans. Here, isotopes like Fluorine-18 are employed to help visualize metabolic processes in the body, assisting doctors in diagnosing various conditions.
- Cancer Treatment: Radioactive isotopes like Iodine-131 are utilized in treating certain types of cancer, particularly thyroid cancer. The isotope targets cancerous cells and helps in reducing tumor size, showcasing the therapeutic potential of isotopes.
- Radiocarbon Dating: Carbon-14 plays an essential role in archeology and geology. It enables scientists to determine the age of ancient artifacts and fossils, thus providing insights into our past and the time frame of evolution.
- Nuclear Energy: In the realm of energy production, isotopes such as Uranium-235 are critical in nuclear reactors. They undergo fission reactions to release energy, highlighting their role in the generation of electricity.
Isotopes are indispensable tools in science and technology, and their significance cannot be overstated. As we continue to explore the atomic world, the understanding and applications of isotopes will likely expand, influencing numerous sectors and driving forward technological advancements.
Understanding isotopes is key to both fundamental science and practical applications in our everyday lives.
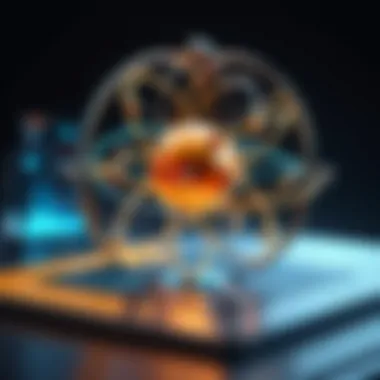
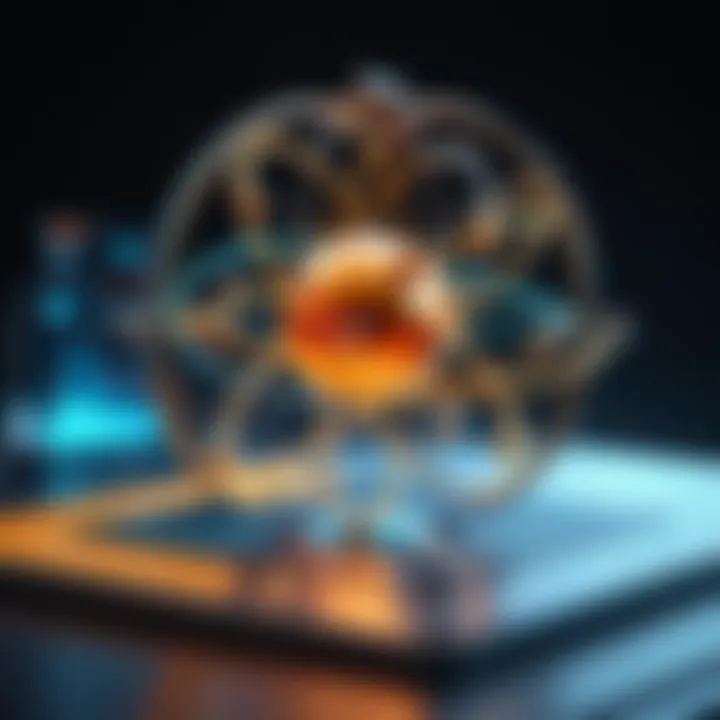
For more detailed information on isotopes, you can visit Wikipedia or Britannica.
In summary, isotopes are more than just a scientific curiosity. They serve as important elements that impact various aspects of society, science, and industry.
Atomic Bonding
Atomic bonding is crucial to understanding the behavior and interactions of various elements in chemistry. It defines how atoms connect with each other to form molecules and compounds. The nature of these bonds influences the properties of materials, their reactivity, and their place in biological systems. Knowledge of atomic bonding enhances comprehension in fields like materials science, biology, and technology, allowing for informed decisions in research and applications.
Ionic Bonds
Ionic bonds are formed through the electrostatic attraction between positively and negatively charged ions. When atoms transfer electrons from one to another, this results in one atom becoming a cation and the other an anion. A classic example of ionic bonding is seen in sodium chloride, where sodium donates an electron to chlorine.
- Properties of Ionic Bonds
Ionic compounds generally exhibit high melting and boiling points. These compounds also tend to dissolve well in polar solvents like water. However, they do not conduct electricity in solid form; they only do so when melted or dissolved in water.
The strength of an ionic bond is influenced by the charge of the ions and their sizes. Greater charge often leads to stronger bonds. Understanding this bonding is vital for industries relying on materials with specific properties, such as ceramics and fertilizers.
Covalent Bonds
Covalent bonds are formed when two atoms share electrons, typically seen in non-metal elements. This type of bonding can result in the formation of molecules. For instance, the water molecule consists of two hydrogen atoms covalently bonded to one oxygen atom.
- Types of Covalent Bonds
- Single Bonds: Involving the sharing of one pair of electrons.
- Double Bonds: Involving two pairs of electrons.
- Triple Bonds: Involving three pairs of electrons.
Covalent bonds hold significant importance in biological systems. Molecules such as DNA and proteins are structured through covalent bonding. These bonds tend to have lower melting points compared to ionic bonds and are not as soluble in water. However, covalent networks, like diamond, have extraordinary hardness.
Metallic Bonds
Metallic bonds occur between metal atoms and are characterized by a 'sea of electrons' that are free to move around. This model explains many properties of metals, such as conductivity and malleability. The delocalized electrons allow for electrical conductivity, as they can carry charge throughout the metal lattice.
- Properties of Metallic Bonds
- Conductivity: Metals conduct electricity and heat efficiently.
- Malleability and Ductility: Metals can be shaped into sheets or drawn into wires without breaking.
- Luster: Metallic bonds account for the shiny appearance of metals.
The understanding of metallic bonds is fundamental for industries such as electronics and construction, where the materials' electrical and physical properties are paramount.
Atomic bonding shapes both the microscopic world of atoms and the macroscopic properties of materials, influencing everything from chemical reactions to everyday applications in technology and industry.
Modern Technologies and Atomic Structure
Understanding atomic structure has become increasingly relevant in the modern technological landscape. Recent advances in science hinge on the manipulation and comprehension of atomic behavior. This section highlights how breakthroughs in atomic research inform various contemporary applications. By decoding the properties of atoms, scientists and engineers harness their potential for innovations.
Additionally, atomic structure underpins essential aspects of developments in nanotechnology and computing. Each subpoint illustrates the vast possibilities stemming from atomic manipulation and its implications for industries and daily life.
Applications in Nanotechnology
Nanotechnology leverages atomic and molecular components to create materials and devices at nanoscale, typically between 1 to 100 nanometers. At this scale, materials exhibit unique properties due to quantum effects, which cannot be observed in bulk materials. These properties lead to numerous applications, including:
- Enhanced Material Strength: Understanding atomic interactions allows for the creation of lighter and stronger materials. Carbon nanotubes are one prime example, significantly more durable than steel.
- Improved Catalysis: Nanostructured catalysts can increase reaction rates in chemical processes. This has implications for energy conversion and environmental mitigation through more efficient reactions.
- Medical Applications: Nanotechnology aids in drug delivery systems by enabling more efficient targeting of specific cells, reducing side effects associated with traditional therapies.
Innovations in this field, driven by knowledge of atomic structure, suggest a future where materials can be specifically engineered for desired functions. Thus, atomic insights are crucial in driving forward nanotechnology.
Implications in Computing
Atomic structure plays a pivotal role in the realm of computing. As electronic devices become more sophisticated, the desire for smaller and faster components grows. Quantum mechanics and atomic principles are central to this field. Notable implications include:
- Semiconductor Technology: The foundation of modern electronics relies on the understanding of atomic structure to create semiconductors. Devices such as transistors and diodes depend on manipulating atomic properties of silicon and other materials.
- Quantum Computing: Quantum computing embraces the principles of quantum mechanics, using qubits that can exist in multiple states at once. This development surpasses traditional computing capabilities, promising exponential increases in processing power for complex tasks.
- Data Storage Solutions: Atomic-scale data storage technologies offer the possibility of storing vast amounts of information in smaller spaces. Techniques like atomic-level manipulation of magnetic states are being developed for high-density memory solutions.
As a result, understanding atomic structure not only enhances current technologies but also carves new avenues in computing, making this knowledge indispensable for future innovations.
"The study of atomic structure is not just fundamental for theoretical physics but is essential for technological advancement in today's world."
In summary, the exploration of atomic structure opens up transformative possibilities for modern technologies, particularly in nanotechnology and computing. These domains illustrate how fundamental scientific knowledge translates into practical applications that shape our technological future.
Ending
The conclusion of this article serves as a critical summation of the entire discussion surrounding atomic structure. It encapsulates the key concepts that have been presented while emphasizing their significance in both scientific understanding and practical applications.
Recap of Atomic Structure
In reviewing the structure of the atom, it is essential to highlight the fundamental components: protons, neutrons, and electrons. Protons bear a positive charge and are located within the nucleus. Neutrons, being neutral, also reside in the nucleus, contributing to the atom's mass stability. Electrons, with their negative charge, are dispersed in energy levels surrounding the nucleus, forming what is often conceptualized as an electron cloud. Each of these particles plays a unique role in defining the properties of an element. This recap is vital, as it not only reinforces the individual contributions of these particles but also illustrates how their interactions establish the diverse range of matter we observe in the universe.
Future Directions in Atomic Research
Looking ahead, the future of atomic research is poised for significant advancements. The convergence of quantum mechanics with emerging technologies like nanotechnology and computing heralds new possibilities. For instance, improving the manipulation of atomic structures could unlock enhanced materials with tailored properties.
Additionally, the ongoing exploration of isotopic applications in medicine, particularly in targeted therapies and diagnostics, remains a promising avenue of research. Understanding the implications of atomic behavior will continue to be essential as we delve deeper into both theoretical physics and practical applications.
"Research into atomic structure remains at the forefront of scientific inquiry, integrating disciplines and pushing the boundaries of knowledge."