Exploring the Types of MOSFETs: A Comprehensive Guide
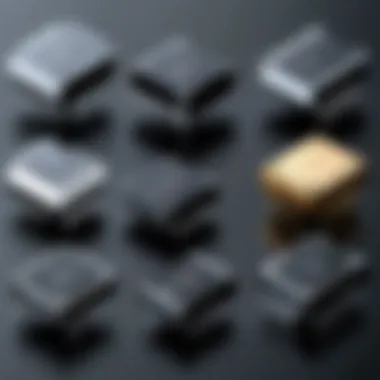
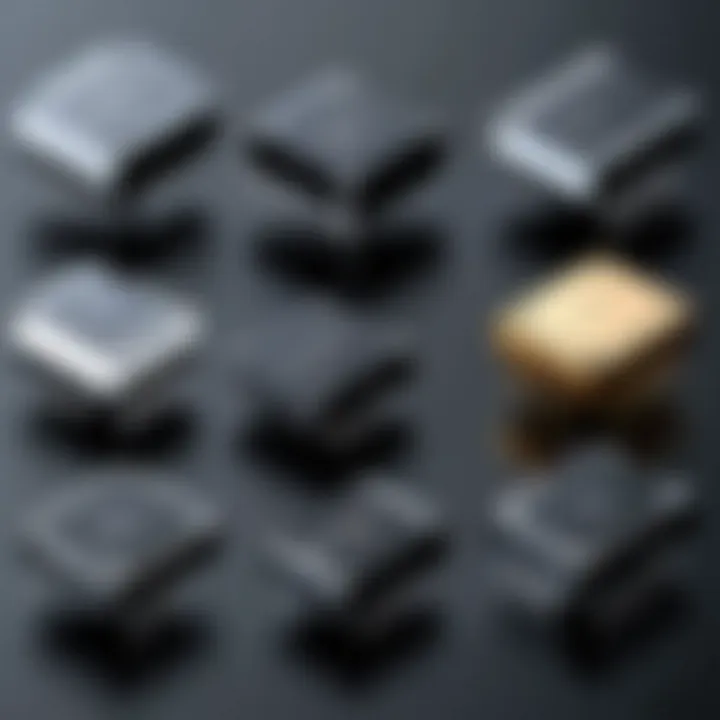
Overview of Topic
Understanding Metal-Oxide-Semiconductor Field-Effect Transistors, or MOSFETs, is crucial for anyone involved in electronics today. These components play a significant role in managing electrical signals. By controlling current flow, they serve various functions in devices ranging from simple light switches to complex microprocessors.
This article focuses on the different types of MOSFETs, emphasizing their unique characteristics, operational principles, and applications. It aims to enhance the reader's knowledge, which is essential for both academic pursuits and practical applications in the tech field.
Historically, MOSFET technology emerged from developments in semiconductor physics in the 1960s. Over the decades, they have evolved significantly, making them a cornerstone of modern electronic devices.
Fundamentals Explained
At its core, a MOSFET is a type of transistor that uses voltage to control the flow of current. It consists of three terminals: gate, drain, and source. The key principles behind MOSFET operation involve controlling the conductivity of a channel through which current flows in response to an electric field applied at the gate terminal.
Key Terms and Definitions
- Transistor: A semiconductor device used to amplify or switch electronic signals.
- Channel: The region between the source and drain through which current flows.
- Gate Voltage: The voltage applied at the gate terminal that controls the conductivity of the channel.
Basic concepts are essential. Understanding MOSFETs requires grasping how they differ from bipolar junction transistors (BJTs), mainly in their voltage-driven operation versus current-driven operation.
Practical Applications and Examples
One of the most notable applications of MOSFETs lies in power management. They are commonly found in switching power supplies and electric vehicles, where efficient power conversion is crucial.
Real-World Examples
- In computer CPUs, MOSFETs manage the on/off states of logic gates, enabling complex calculations.
- In audio amplifiers, they help control the output to speakers, ensuring quality sound without distortion.
Hands-on projects may involve creating basic circuits with MOSFETs, like dimming an LED or controlling a motor.
Sample Implementation
Advanced Topics and Latest Trends
The field of MOSFET technology is continually advancing. Recent trends focus on enhancing the efficiency and speed of these components. Researchers are exploring new materials, such as gallium nitride, to improve performance.
Advanced techniques include the development of high-voltage MOSFETs and ultra-low on-resistance designs. These innovations help reduce energy loss in applications like renewable energy systems and electric vehicles.
Tips and Resources for Further Learning
To delve deeper into MOSFET technology, consider exploring resources like:
- Books: "Microelectronic Circuits" by Sedra and Smith.
- Online Courses: Platforms like Coursera and edX offer courses on semiconductor devices.
- Forums: Websites like Reddit have communities focused on electronics, providing real-world insights and assistance.
You can also refer to Wikipedia and Britannica for foundational knowledge and historical context regarding MOSFETs, as they host a wealth of information on electrical engineering topics.
Intro to MOSFETs
Metal-Oxide-Semiconductor Field-Effect Transistors, commonly known as MOSFETs, are essential components in modern electronics. Their significance lies in the ability to control electrical signals efficiently, which is critical in various applications ranging from simple switches to complex integrated circuits. Understanding the fundamental aspects of MOSFETs will help readers appreciate their role and functionality in electronic systems.
Definition and Overview
A MOSFET is a type of transistor that is used to amplify or switch electronic signals. It consists of three main terminals: the gate, the drain, and the source. The gate is insulated from the channel by a thin layer of oxide, typically silicon dioxide. This structure allows the gate to control the flow of current between the source and drain terminals without a direct electrical connection. There are two primary types of MOSFETs: enhancement mode and depletion mode. Enhancement mode devices are off when no voltage is applied to the gate, while depletion mode devices can be considered normally on and require a gate voltage to turn off.
Importance in Modern Electronics
MOSFETs play a pivotal role in the functionality of digital and analog circuits. They have become the backbone of many electronic devices due to their fast switching speeds and high efficiency. In digital circuits, for example, MOSFETs are used in logic gates, which are fundamental to computer operations. Additionally, in power electronics, they control the flow of electrical power in applications such as motor drives, power supplies, and inverters.
"MOSFETs are integral to the advancement of compact and efficient electronic designs."
The flexibility of MOSFETs in various applications makes them indispensable. Their low power consumption and high-density integration capabilities enable the development of smaller and more powerful devices. Understanding the characteristics and types of MOSFETs is crucial for anyone involved in electronics, providing insights that can facilitate better design and innovation in the field.
Basic Structure of MOSFETs
Understanding the basic structure of Metal-Oxide-Semiconductor Field-Effect Transistors (MOSFETs) is pivotal for grasping their functionality and applications. The structure determines how the device operates and how efficiently it performs in various electronic circuits. Recognizing these aspects is essential for anyone looking to work with or understand modern electronics.
Key Components
A MOSFET consists of several key components that work together to control the flow of current. These components include:
- Source: This terminal allows current to enter the device. In an N-channel MOSFET, the source is typically connected to a voltage that refers to the ground level.
- Drain: The drain is where the current exits the MOSFET. The voltage at the drain can influence the behavior of the device.
- Gate: The gate terminal controls the MOSFET. It is insulated from the channel by a thin layer of oxide, allowing a voltage applied here to create an electric field that influences current flow.
- Body (or Substrate): The body serves as the foundation for the device, linking all components. It impacts the MOSFET’s performance, particularly under certain operational conditions.
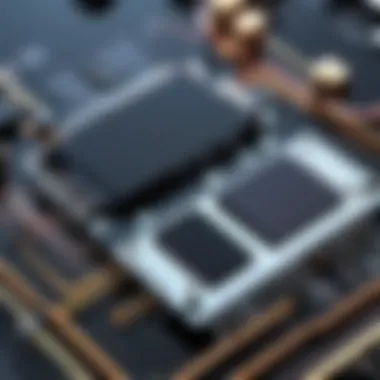
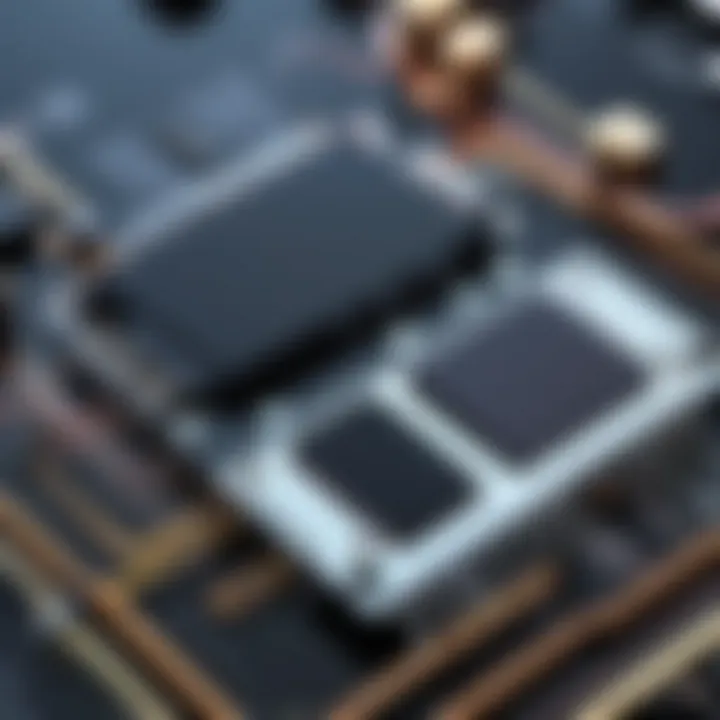
Each component plays a vital role in ensuring the MOSFET operates correctly and efficiently.
Operational Principle
The operational principle of MOSFETs centers around the control of the electric field. When a voltage is applied to the gate, an electric field forms in the channel region, which is situated between the source and drain. This field can attract or repel charge carriers, thereby facilitating or obstructing the flow of current.
- Enhancement Mode: In enhancement mode MOSFETs, a voltage at the gate creates a layer of conductive material in the channel when there wasn’t any initially. This allows for current to flow from the source to the drain.
- Depletion Mode: Conversely, in depletion mode devices, applying a negative voltage to the gate depletes the channel of charge carriers, creating a high-resistance state, thus stopping current flow.
These principles enable MOSFETs to serve as switches or amplifiers in circuits, making them an invaluable component in electronic applications.
Efficient understanding of these components and operational principles is key to leveraging MOSFET technology effectively in various applications.
In summary, comprehending the basic structure and operational principles of MOSFETs enhances the ability to utilize these components effectively in design and applications spanning different fields. The knowledge gained will serve as a foundation for deeper exploration into the numerous types of MOSFETs and their specific utility in our modern technological landscape.
Types of MOSFETs
The classification of Metal-Oxide-Semiconductor Field-Effect Transistors (MOSFETs) plays a critical role in understanding their diverse applications. Each type presents unique characteristics that dictate their functionality in circuits. Recognizing the distinctions between these MOSFET types allows engineers and designers to make informed decisions in selecting appropriate components for specific electronic tasks.
In this section, we will explore the major types of MOSFETs—Enhancement Mode, Depletion Mode, N-Channel, and P-Channel—each demonstrating particular features suitable for varying applications and performance outcomes. Through this exploration, readers can grasp the fundamental operational principles guiding each type of MOSFET and their practical significance in electronics.
Enhancement Mode MOSFETs
Enhancement Mode MOSFETs are predominantly utilized in electronic applications requiring switching. They operate by increasing the conductivity of the channel when a specific voltage is applied to the gate electrode. In this mode, when the gate voltage surpasses the threshold voltage, an inversion layer forms, allowing current to flow between the drain and source. This feature makes enhancement mode devices particularly favorable in digital circuits where a strong ON/OFF characteristic is necessary.
Key benefits of Enhancement Mode MOSFETs include:
- High input impedance
- Low power consumption
- Greater switching speed
However, considerations should be taken into account, especially in terms of gate voltage specifications and thermal management during operation. Their robust performance under these conditions makes them essential in contemporary electronic design.
Depletion Mode MOSFETs
Depletion Mode MOSFETs, in contrast, operate by reducing the conductivity of the channel when a negative gate voltage is applied. They are unique because they can conduct current even without biasing the gate. This allows for the control of current flow, effectively enabling them to function as a switch or amplifier when required.
The benefits of Depletion Mode MOSFETs can be summarized as:
- Can operate in normally ON state
- Useful in analog applications
- Provide high-frequency performance
Despite these advantages, designers should be aware of their more complex biasing requirements, which can complicate circuit designs. Their characteristics make them suitable for specific applications such as RF amplifiers or analog signal processing, where their unique ability to control current flow is beneficial.
N-Channel MOSFETs
N-Channel MOSFETs are one of the most common types employed in electronic circuits. They utilize n-type semiconductor material for the channel, allowing electrons to be the primary charge carriers. These devices have lower on-resistance than their P-Channel counterparts, enabling higher efficiency and reduced power loss. Furthermore, they typically deliver greater current handling capabilities, which is vital for power applications.
Advantages of N-Channel MOSFETs include:
- Superior performance in high-frequency applications
- Efficient operation with lower power dissipation
- Simpler drive requirements compared to P-Channel versions
When implementing N-Channel MOSFETs, it is crucial to ensure proper gate drive voltages, as this can significantly affect performance and reliability.
P-Channel MOSFETs
P-Channel MOSFETs function through p-type semiconductor materials where holes serve as charge carriers. These transistors are often used in applications that require high-side switching or in circuits where negative-ground configurations are prevalent. Due to their characteristics, P-Channel devices typically have higher on-resistance, making them less efficient for high current applications when compared to N-Channel types.
Some notable aspects of P-Channel MOSFETs are:
- Convenient in low-side switching applications
- Better suited for specific circuit configurations, like load switches
- Key role in complementary push-pull stages
Users should think about thermal considerations and proper design when using P-Channel MOSFETs, particularly in reducing power dissipation and maintaining efficiency.
In brief, understanding different types of MOSFETs provides valuable insights into designing effective electronic circuits. With their distinct characteristics, Enhancement Mode, Depletion Mode, N-Channel, and P-Channel devices each present unique advantages and considerations that help engineers tailor solutions for specific electronic needs.
Application Areas of MOSFETs
MOSFETs play a critical role in various application areas within electronics. Understanding these areas helps us see the broader relevance of this technology in both current and emerging markets. Evaluating their application capabilities can lead to better designs and more efficient systems. This section outlines significant fields where MOSFETs are utilized.
Power Electronics
Power electronics is a major application area for MOSFETs. These devices are essential in controlling and converting electrical power efficiently. MOSFETs can handle high voltages and currents, making them suitable for power supplies, inverters, and converters. Their low on-resistance allows better thermal management and minimizes energy loss.
The ability of MOSFETs to switch rapidly enhances their utility in various applications. For example, in renewable energy systems, like solar inverters, MOSFETs help optimize energy conversion processes. Additionally, they provide better efficiency in electric vehicles and industrial automation.
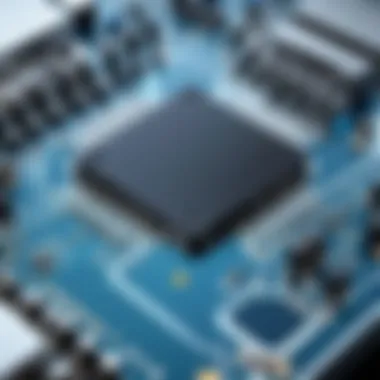
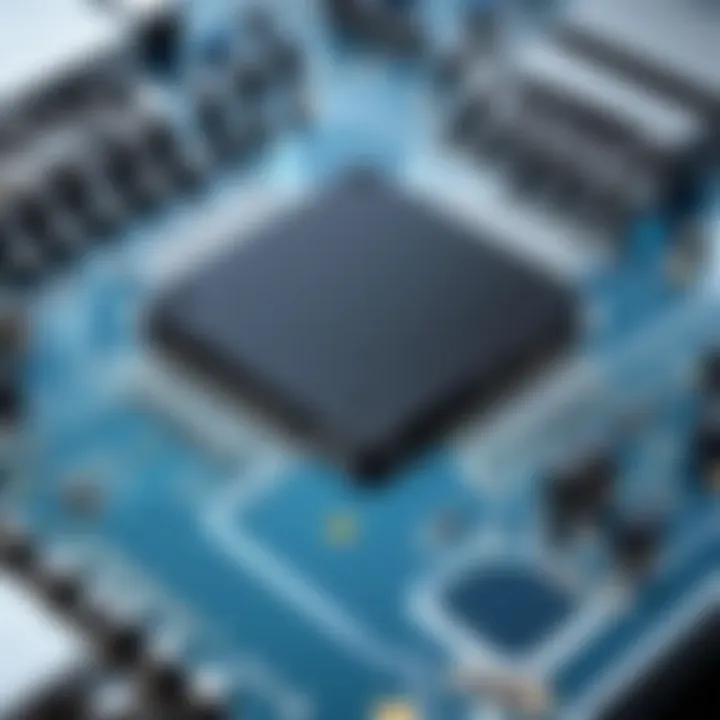
Signal Amplification
In the realm of signal amplification, MOSFETs are highly valued for their exceptional gain properties. They can amplify weak signals without significant distortion, essential for communication systems. MOSFET amplifiers are widely used in audio equipment, radio frequency devices, and more.
Their high input impedance is a crucial characteristic that allows for minimal loading on previous stages of circuits. This leads to cleaner signal processing and improved performance in various electronic devices. Different configurations, such as common source and common drain, can be employed depending on the needs of the application.
Digital Circuits
Digital circuits rely heavily on MOSFET technology. These transistors form the backbone of modern computing and digital logic design. Since MOSFETs can represent binary states, they are integral in constructing logic gates and flip-flops.
The scalability of MOSFETs is a significant advantage, enabling manufacturers to produce smaller and more powerful chips. This miniaturization is vital as the demand for compact and efficient devices continues to grow. Furthermore, advancements in CMOS technology capitalize on the unique properties of MOSFETs, enhancing performance and energy efficiency in digital applications.
Switching Applications
Switching applications also benefit significantly from MOSFET technology. They are commonly used in electronic switches that control the flow of electricity in various devices. The ability to switch on and off rapidly translates to better control over power delivery.
In power management systems, efficiency is paramount. MOSFETs excel in this area, where they allow for precision in controlling electrical loads. Applications range from simple relays to complex motor controllers. The versatility of MOSFETs in switching applications contributes to innovations in consumer electronics, industrial machinery, and high-frequency trading systems.
"The application of MOSFETs stretches across various fields, emphasizing their significance in powering today’s electronic landscape."
The exploration of these application areas illustrates the critical role that MOSFETs play in advancing technology. Understanding how they function in these different contexts is essential for anyone interested in electronics.
Characteristics of Different MOSFET Types
The characteristics of different MOSFET types are critical to understanding their performance and suitability for various applications. Each characteristic offers unique insights into how the MOSFET functions under certain conditions. By examining these traits, engineers and designers can make informed decisions about component selection and system design. This section will elaborate on four fundamental characteristics: threshold voltage, transconductance, on-resistance, and switching speed, their implications, and their significance in the broader context of MOSFET technology.
Threshold Voltage
Threshold voltage is the minimum gate-to-source voltage that must be applied to a MOSFET to turn it on and allow current to flow. It is a fundamental parameter as it influences how the device behaves during operation. A lower threshold voltage can lead to easier device activation, which can be advantageous in low-voltage applications.
Factors that can affect threshold voltage include:
- Temperature: Increasing the temperature often lowers the threshold voltage.
- Fabrication process: Variations in the manufacturing process can lead to differences in threshold values.
- Device geometry: The size and design of the MOSFET can influence overall performance.
The threshold voltage is pivotal for designing circuits where precise control of current flow is necessary. Designers must consider this to optimize efficiency.
Transconductance
Transconductance, often denoted as gm, is a parameter that measures the effectiveness of a MOSFET in controlling the output current based on a change in gate voltage. A higher transconductance value indicates that a small change in the input voltage can lead to larger changes in output current.
Benefits of a high transconductance include:
- Enhanced amplification capabilities in analog applications.
- Improved switching behavior for digital circuits, which can lead to faster performance.
Engineers often assess transconductance during the design stage to ensure that the MOSFET will meet performance requirements under expected operating conditions.
On-Resistance
On-resistance, symbolized by R_on, represents the resistance between the drain and source terminals when the MOSFET is in the ON state. A lower on-resistance is generally desirable, as it minimizes power loss and heat generation during operation. The significance of on-resistance can be seen in:
- Power efficiency: Lower R_on leads to less energy wasted as heat.
- Thermal management: Reduced on-resistance can simplify thermal design considerations, reducing the need for extensive cooling solutions.
It is crucial to evaluate the on-resistance figures when integrating MOSFETs into high-power applications, such as power supplies and motor drives.
Switching Speed
Switching speed defines how quickly a MOSFET can transition between its ON and OFF states. This characteristic is important for pulse applications, digital logic, and RF amplifiers where fast switching contributes to overall system performance. Factors influencing switching speed include:
- Gate charge: A lower gate charge often leads to faster switching times.
- Capacitance effects: Input capacitance can limit the response time of the device to changes in input signals.
Fast switching speeds allow for greater efficiency and performance in electronic circuits. As demands for higher data rates rise in communication technologies, the emphasis on switching speed will continue to grow.
Comparison of MOSFET Types
The comparison of different types of MOSFETs is crucial for understanding their diverse functionalities and applications. Each type of MOSFET has its own characteristics and suitable use cases, which may affect performance, efficiency, and design choices in electronic circuits. This section highlights these differences clearly. An informed comparison enables engineers and designers to select the appropriate type for their specific requirements, ensuring optimal functionality.
N-Channel vs P-Channel
N-Channel and P-Channel MOSFETs represent two foundational categories of field-effect transistors.
N-Channel MOSFETs: These are often preferred for high-speed and power applications. An N-Channel MOSFET allows electrons to flow from the source to the drain when a sufficient voltage is applied to the gate. This type usually exhibits lower on-resistance, which means they are more efficient in conducting current. They are widely used in applications like switching power supplies, motor controllers, and amplification circuits.
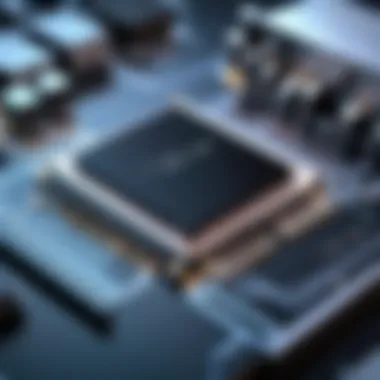
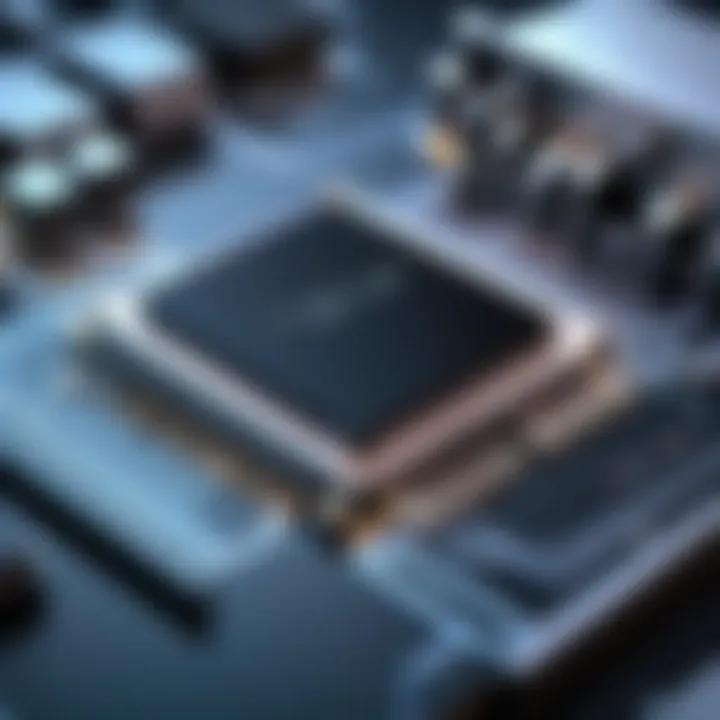
P-Channel MOSFETs: P-Channel MOSFETs work in the opposite manner. They enable the flow of holes from the source to the drain. This behavior makes them typically less efficient than their N-Channel counterparts, particularly for high-current applications. However, they are essential in certain circuit designs where N-Channel devices cannot be easily implemented. P-Channel MOSFETs are often found in low-side switching circuits, as well as complementary push-pull circuits where both types can be used effectively.
"The choice between N-Channel and P-Channel MOSFETs depends largely on the application and desired circuit behavior."
In summary, while N-Channel devices generally offer higher performance, P-Channel devices play a significant role in specific applications, especially when integration within a circuit demands it.
Enhancement vs Depletion
Enhancement mode and depletion mode MOSFETs are two operational principles that define performance characteristics and usability.
Enhancement Mode MOSFETs: In enhancement mode, the MOSFET is normally off when no voltage is applied to the gate. An external voltage is required to enhance the conductive channel, allowing current to flow between source and the drain. This mode is predominant in modern circuits and offers advantages like high input impedance and lower power consumption when off. These transistors are suitable for digital circuits, amplifying applications, and switching devices.
Depletion Mode MOSFETs: Conversely, depletion mode MOSFETs are normally on at zero gate voltage. They require a negative gate voltage to deplete the channel of charge carriers, effectively turning the device off. Depletion mode devices provide a unique advantage in specific applications, such as analog circuits and certain types of switching applications. They can maintain a steady current flow even with zero gate voltage, which can be beneficial in certain scenarios.
Recent Developments in MOSFET Technology
The field of Metal-Oxide-Semiconductor Field-Effect Transistors (MOSFETs) is continually advancing. Recent developments have brought new techniques and materials that enhance performance and efficiency. This section explores the innovations that are shaping the MOSFET landscape. Understanding these developments is crucial for those engaged in electronics and related fields. The emphasis will be on two primary areas: materials innovation and design advancements.
Innovations in Materials
One significant advancement in MOSFET technology is the introduction of new materials. Traditionally, silicon has been the dominant semiconductor material used in MOSFETs. However, research has led to the exploration of alternative materials such as gallium nitride (GaN) and silicon carbide (SiC). These materials offer several advantages over silicon. They have higher critical electric fields and greater thermal conductivity, which allows for efficient operation under high power conditions.
The benefits of these innovations include:
- Higher efficiency: GaN and SiC MOSFETs can handle higher voltages and currents, leading to reduced switching losses.
- Improved thermal management: Better heat dissipation capabilities decrease the risk of overheating in high-performance applications.
- Smaller sizes: Devices made from these materials can be smaller, contributing to more compact electronic designs.
As a result, industries such as automotive, renewable energy, and telecommunications are increasingly adopting GaN and SiC MOSFETs.
"The future of MOSFET technology is not only about performance; it’s about how these advancements can change the landscape of power electronics."
Advancements in Design
Alongside material innovations, advancements in MOSFET design are also reshaping the field. New architectures, such as the double-gate MOSFET and FinFET (Fin Field Effect Transistor), have emerged. These designs enhance transistor performance by reducing short-channel effects that can occur in traditional MOSFETs.
Key design advancements include:
- Multigate structures: Enhanced electrostatic control of the channel results in lower leakage currents and improved switching characteristics.
- Integration with other technologies: Combining MOSFETs with complementary devices allows for more energy-efficient circuits.
- Tailored parameters: Specific designs can be optimized for a variety of applications, making them suitable for niche markets in power regulation and signal processing.
In summary, recent developments in materials and design are pivotal for the evolution of MOSFET technology. As these innovations continue to progress, they will drive performance improvements, allowing for wider application and better integration in modern electronic systems.
Future Trends in MOSFET Development
The evolution of Metal-Oxide-Semiconductor Field-Effect Transistors (MOSFETs) continues to accelerate, driven by the advancing needs of modern electronics. Understanding these trends is essential for students, IT professionals, and those exploring programming languages. New developments are not just about performance; they also address efficiency, sustainability, and integration with emerging technologies.
Integration with Other Technologies
In recent years, there has been a strong emphasis on integrating MOSFET technology with other electronic components and systems. This convergence leads to more efficient designs that can streamline operations. For example, when paired with complementary metal-oxide-semiconductor (CMOS) technology, MOSFETs can enhance power efficiency and reduce chip size.
- Synergy with AI Components: The rise of artificial intelligence demands faster processing speeds. When integrated, MOSFETs can help achieve lower latency in processing tasks.
- Collaboration with Photonics: Light-based technologies are growing in prominence. The interplay between optical components and MOSFETs can enable innovative solutions for communications and imaging applications.
- Smart Grids: As energy management grows in importance, MOSFETs are being integrated into smart grid technologies, optimizing power distribution and consumption.
These integrations lead to benefits such as improved thermal management, reduced energy consumption, and enhanced overall performance.
Emerging Applications
As MOSFET technology continues to develop, new applications emerge. This section discusses some of the most notable areas where MOSFETs are being applied beyond traditional usage.
- Electric Vehicles (EVs): The electric vehicle market is expanding rapidly. MOSFETs play a crucial role in power management systems within EVs, enabling efficient conversion and utilization of energy.
- Renewable Energy: Solar inverters utilizing MOSFET technology enhance the conversion efficiency of solar energy into usable power. This application addresses the global shift towards renewable resources.
- Internet of Things (IoT): As IoT devices proliferate, the need for compact, energy-efficient components rises. MOSFETs help meet these requirements while facilitating smart homes and cities.
- Wearables: The demand for smaller, efficient devices in the wearable technology space pushes for innovations in MOSFET designs, focusing on minimizing size without sacrificing performance.
Understanding these emerging applications of MOSFETs not only highlights their versatility but also demonstrates their integral role in shaping the future of technology.
Finale
The conclusion of this article emphasizes the importance of understanding the various types of MOSFETs in today's electronic landscape. As technology evolves, the demand for efficient and reliable components is ever increasing. In this guide, we have explored the distinctive characteristics, applications, and operational principles of different MOSFET types. This deep dive equips readers with valuable insights that are essential for both academic pursuits and practical implementations in electronics.
Understanding the nuances of Enhancement Mode MOSFETs, Depletion Mode MOSFETs, N-Channel, and P-Channel MOSFETs helps in choosing the right device for specific applications. Moreover, recognizing their strengths, weaknesses, and the recent developments in MOSFET technology highlights the relevance of this knowledge in future projects. As we navigate various application areas like power electronics and switching applications, the implications of efficient MOSFET deployment become clearer.
In summary, the knowledge acquired from this article will undoubtedly benefit individuals in their pursuit of designing and implementing more sophisticated electronic systems. The importance of integrating updated technologies into educational programs cannot be overstated as it prepares future professionals for the challenges of a rapidly evolving sector.
Recap of Key Points
- The article provides a comprehensive overview of MOSFET types, namely Enhancement Mode and Depletion Mode MOSFETs, as well as N-Channel and P-Channel varieties.
- Each type carries distinct characteristics that influence their performance in various applications, from signal amplification to digital circuits.
- Recent innovations in materials and design offer potential advancements in MOSFET technology, increasing efficiency and reliability in electronic systems.
- As the electronics industry increasingly relies on MOSFETs, understanding their functionalities and nuances is vital for engineers and students alike.
Implications for Future Research
As we look to the future, further research into MOSFET technology will be critical. The implications extend beyond mere component design. Future studies may focus on:
- Integration with emerging technologies: Investigating how MOSFETs can interact with newer materials such as graphene or silicon carbide for improved thermal and electrical performance.
- Enhanced functionality: Research could explore combining MOSFETs with other semiconductor devices to enable more compact and efficient circuitry.
- Sustainability: Developing more eco-friendly manufacturing processes or recyclable MOSFETs could align with global sustainability goals.
This opens up a plethora of opportunities for innovation and improvement within the electronics field, suggesting that MOSFET research will play a pivotal role in shaping the future of technology.