Understanding Types of Semiconductors and Their Applications
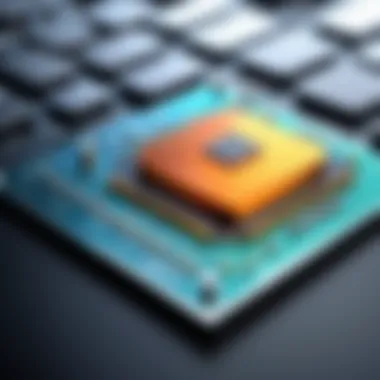
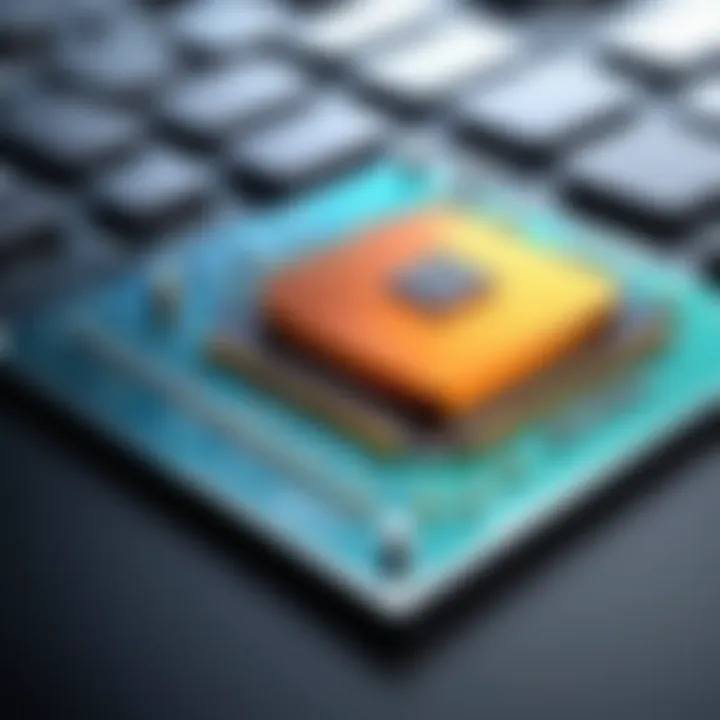
Overview of Topic
Prelude to the main concept covered
Semiconductors are crucial components in modern technology. They are materials whose electrical conductivity lies between that of a conductor and an insulator. This unique property enables semiconductors to control electrical flow, making them essential for countless electronic devices.
Scope and significance in the tech industry
The significance of semiconductors cannot be overstated. They form the backbone of modern electronics. Without them, devices like smartphones, computers, and televisions would not function. Furthermore, the demand for semiconductors is rapidly increasing due to advancements in artificial intelligence, telecommunications, and renewable energy technologies.
Brief history and evolution
The concept of semiconductors has evolved over the years. Early discoveries date back to the 19th century with elements like selenium and silicon. However, the real breakthrough came with the invention of the transistor in the late 1940s, revolutionizing the electronics industry. Since then, semiconductors have continuously evolved, leading to the development of integrated circuits in the 1960s and more recent advancements in nanoelectronics.
Fundamentals Explained
Core principles and theories related to the topic
At the heart of semiconductor functionality is the behavior of electrons in a material. Semiconductor materials can be tailored to either hold or release electrons, depending on their allowed energy levels. This makes it possible to create p-type and n-type semiconductors—each type possessing distinct conductivity properties.
Key terminology and definitions
Several terms are fundamental to understanding semiconductors:
- P-type semiconductor: Contains holes, allowing electrical current to flow through absence of electrons.
- N-type semiconductor: Contains extra electrons, enhancing electrical conductivity.
- Doping: The process of adding impurities to a semiconductor to change its conductivity.
Basic concepts and foundational knowledge
A good understanding of semiconductors begins with awareness of how they can operate in different conditions. For instance, temperature changes can affect semiconductor properties. In higher temperatures, more charge carriers (electrons or holes) can be generated, impacting the overall conductivity. Key graphs, such as the energy band diagram, provide deeper insight into this phenomenon.
Practical Applications and Examples
Real-world case studies and applications
Semiconductors are used in diverse applications:
- Microprocessors: Found in computers and smartphones.
- LEDs: Used in lighting solutions and displays, showcasing the efficiency of semiconductor materials.
- Solar Cells: Use semiconductors to convert sunlight into electricity effectively.
Demonstrations and hands-on projects
Those interested in practical exploration can start with simple projects using common chips. You can model a basic transistor circuit on a breadboard, lighting an LED with minimal components.
Code snippets and implementation guidelines
For programming-related applications, understanding how to read sensor data via microcontrollers is crucial. Incorporating semiconductor components with code can lead to various devices, from simple LEDs but depending on the behavior of sensors like semiconductors often used in temperature detection or pressure monitoring.
Advanced Topics and Latest Trends
Cutting-edge developments in the field
Currently, research focuses on the development of two-dimensional materials, which have extraordinary properties. For example, Graphene exhibits excellent conductivity, offering a glimpse into the future of ultra-fast electronics.
Advanced techniques and methodologies
Innovation continues with manufacturing techniques, such as atomic layer deposition (ALD) enhancing the precision at which semiconductor elements are created. The goal is to minimize size while maximizing performance.
Future prospects and upcoming trends
The semiconductor industry is on the verge of transformative changes. Quantum computing and increasingly complex sensor technologies are right on the horizon. These advancements are characterized not only by improving performance but also by addressing energy consumption concerns crucial to environmental sustainability.
Tips and Resources for Further Learning
Recommended books, courses, and online resources
- Semiconductor Physics and Devices by Donald A. Neamen
- Microelectronic Circuits by Adel S. Sedra and Kenneth C. Smith
- Online platforms like Coursera or edX provide valuable courses on electronics and semiconductor devices.
Tools and software for practical usage
Utilizing software such as LTSpice for simulation and schematic design can enhance understanding of semiconductor circuits. Other tools like Arduino and Raspberry Pi help integrate semiconductors into hands-on projects, linking theoretical concepts with practical applications.
To summarize, semiconductors play a vital role in modern technology, impacting numerous fields. Understanding their fundamentals, applications, and future trends is essential for professionals and aspiring learners alike.
Foreword to Semiconductors
Semiconductors play an essential role in the realm of modern electronics. They form the backbone of countless devices that people use daily. From smartphones to computers, semiconductors are critical components that enable various functionalities, making them indispensable in today's technology landscape.
In essence, a semiconductor is a material with electrical conductivity between that of a conductor and an insulator. This ability to control electrical flow is what makes semicondutors so versatile. Factors such as temperature and doping with specific materials can alter their conductive behavior. This flexibility allows for a range of applications from simple diodes to complex microprocessors.
The advantages of semiconductors include:
- Size: Semiconductors can be miniaturized, allowing the creation of compact electronic devices.
- Efficiency: They often perform their functions using less power than traditional components, which is essential for portable technology.
- Scalability: They can be integrated on a large scale, leading to improved performance while maintaining low costs.
Moreover, understanding semiconductors provides insights into key considerations for various electronics applications, including:
- Heat Management: As semiconductors conduct electricity, managing heat becomes crucial since overheating can lead to malfunction.
- Material Selection: Different semiconductor materials such as silicon or gallium arsenide offer unique properties suitable for distinct applications.
- Environmental Impact: Consideration of how semiconductor manufacturing impacts the environment is becoming increasingly relevant in this industry.
Understanding semiconductors is thus critical for those engaged in designing and working with electronic devices. This knowledge lays the groundwork for deeper explorations in classification and specific types of semiconductors, all paving the way for advancements in technology.
Overall, the exploration of semiconductors leads to a more profound comprehension of technology that drives current and future innovations. Those learning programming, students, and IT professionals will find that having a solid grasp of semiconductors is invaluable not only for understanding electronic devices, but also for driving future tech development. This article aims to distill the complexity of these materials into clear insights that resonate with the technically-inclined audience.
Understanding the Semiconductor Material
Semiconductors form the backbone of modern electronics, making it essential to thoroughly understand these materials for anyone venturing into the technology domain. At its core, a semiconductor material possesses electrical conductivity that is between that of an insulator and a conductor. This unique property enables semiconductors to maintain their place in various electronic devices, including diodes, transistors, and integrated circuits.
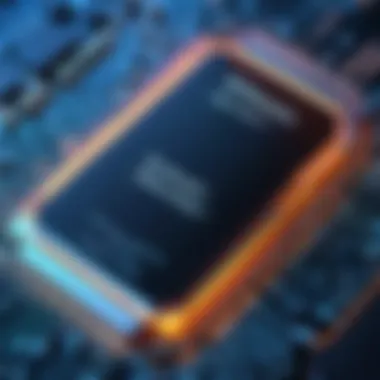
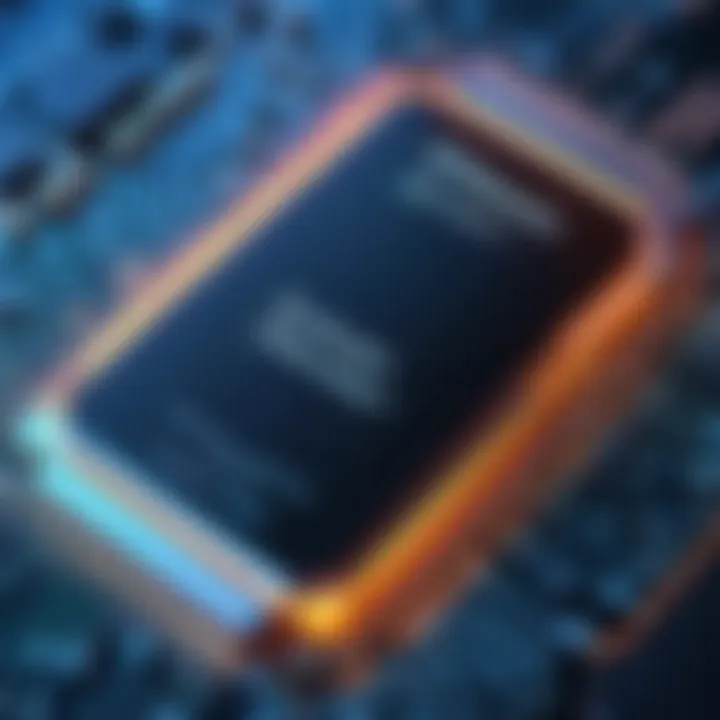
The Role of Atomic Structure
The atomic structure of a semiconductor is crucial. Silicon, the most widely used semiconductor, has four valence electrons. These electrons are responsible for forming bonds in a crystalline lattice structure. When integrated with other elements during production, this structure can modify the material's properties and enhance its functionality. Important for engineering considerations, the simplicity—yet complexity—of silicon profoundly influences development and applications in technology.
Benefits of Studying Semiconductors
A strong grasp of semiconductor materials allows technology professionals to innovate effectively. Understanding how different materials react to impurities can lead to improved efficiency and performance in semiconductors.
Some significant benefits include:
- Enhanced Performance Properties: The selection of specific materials contributes to better operating speed and power savings.
- Economic Impact: Efficient semiconductor manufacturing techniques translate to cost reductions.
- Sustainability: Knowledge of materials contributes to developing eco-friendly solutions, minimizing electronic waste.
In practice, knowledge of semiconductor materials influences everything from product design nuances to optimization of performance. Engineers regularly evaluate the base materials to customize various components, reflecting core application strategies. Through an understanding of semiconductor materials, relevant innovations spring forth:
“The future of technology relies heavily on imroving semiconductor performance and efficiency.”
Considerations in Material Selection
Material selection can dramatically impact device attributes. Factors like thermal conductivity, bandgap, and capacitance play substantial roles. For example, materials used for light-emitting diodes (LEDs) necessitate high efficiency and controlled thermal management to maximize product lifespan and performance.
Critical considerations can include:
- Electrical Conductivity Characteristics: Volumes of carriers in the material governs whether it will exhibit insulating, negative, or positive characteristics based on the doping process.
- Temperature Behavior: Some semiconductors behave differently at varying temperatures, which needs to be comprehensively assessed.
Understanding these attributes shapes decisions across several domains in technology construction and troubleshooting. In tech development, evaluating how different materials operate helps shape the design considerations behind popular devices.
Classification of Semiconductors
The classification of semiconductors is essential for understanding how they function in various applications. It allows for a more organized approach to grasping their physical, chemical, and electronic properties. By categorizing semiconductors into elemental, compound, intrinsic, and extrinsic types, we can identify their respective advantages and disadvantages, and subsequently determine appropriate uses for each. Also, nuances in their capabilities make certain types more suitable for distinct environments or technologies, thus enhancing device performance.
Elemental Semiconductors
Silicon
Silicon is the most widely utilized semiconductor in the industry. Its abundance and effective structural properties resonate with engineers. The most notable characteristic of silicon is its moderate band gap of approximately 1.1 eV, which makes it effective in efficiently converting electrical signals.
The main benefit is in its versatility; silicon can be found in computer chips, photovoltaic cells, and many consumer electronic goods. One unique feature of silicon is its native oxide, silicon dioxide, which effectively confines electric fields and enhances device performance. However, it should be mentioned that silicon shows limitations at higher temperatures, affecting its performance viability.
Germanium
Germanium holds historical significance as an earlier semiconductor in devices such as transistors. Characterized by a smaller band gap of about 0.67 eV, it performs exceptionally in high-frequency and low-noise applications. One key advantage of germanium is its efficiency in photon detection, particularly in optical communication devices.
Nevertheless, germanium’s primary disadvantage lies in cost. It is relatively more expensive compared to silicon. Also, its susceptibility to oxidation can pose challenges during manufacturing processing and material stability over time.
Compound Semiconductors
Gallium Arsenide
Gallium arsenide is notable for applications in optoelectronic devices. With a band gap of 1.43 eV, it allows for efficient light emission thus used in lasers and LED technologies. The key benefit of gallium arsenide over silicon is its higher electron mobility, which means faster switching speeds for devices.
Yet, there are downsides. Gallium arsenide is more costly to process. This inhibits widespread adoption over silicon in less critical applications, as the material may not be value-effective.
Indium Phosphide
Indium phosphide is another crucial compound semiconductor widely used for high-frequency and high-temperature applications. With its wider band gap, measured around 1.34 eV, it excels in optoelectronic components like laser diodes and photodetectors.
The appeal of indium phosphide lies in its superior leakage current performance, which helps in minimizing power loss. A unique feature is its compatibility with monolithic microwave integrated circuit technology, which provides another avenue for applications. Its downsides include scarcity and higher manufacturing costs compared to silicon.
Cadmium Telluride
Cadmium telluride is significant in the realm of solar applications. As a thin-film photovoltaic material, it allows efficient solar energy conversion. It has a band gap of approximately 1.5 eV, ideal for light absorption.
One advantage of cadmium telluride includes its low production cost relative to alternative solar materials. Its performance in various environmental conditions also stands out. However, there are environmental concerns raised, as cadmium is toxic and poses challenges during disposal. This limitation effects public acceptance and technology adoption from regulatory perspectives.
Intrinsic Semiconductors
Definitions
Intrinsic semiconductors are pure materials without significant impurities or dopants. This uniformity caters specifically to their electronic properties, allowing the evaluation of thermal and optoelectronic behavior in a controlled manner. They serve to lay groundwork for understanding complex configurations.
The appeal lyes in their predictable behaviors at varying temperatures. However, the base conductivity of intrinsic semiconductors typically rivals that of higher impurities which leads to utility limitations.
Properties
The primary property of intrinsic semiconductors is their conduction mechanism tied to thermal energy at elevated levels. This drives excited electrons through the material enabling conduction when sufficiently energized. While they retain stability under controlled environments, variability and low inherent conductivity can limit their application in high-efficiency circuits compared to doped semiconductors.
Extrinsic Semiconductors
N-type
N-type semiconductors are formed by introducing donor impurities, usually from groups V elements. Electrons become the primary charge carriers, enhancing current carries efficiency. A defining property is the availability of free electrons that results in increased conductivity. The utility in applications like integrated circuits and photonics arises from their electronic properties.
However, these benefits can come at a cost introduce complexities in behavior at high temperatures, leading to conduction anomalies. Occasional counter-effects that may decrease performance make careful design consideration significant.
P-type
Conversely, P-type semiconductors are made by doping acceptor impurities leading to ‘holes'. These holes serve as positive charge carriers. Similar to N-type, the advantage lies in increased conductivity aspects in applications like diodes and transistors. One key benefit of P-type is complementary devices collaborating with N-type, forming p-n junctions vital in many electronic schemas.
Yet, a tricky point worth mentioning is decreased thermal stability observed in certain configurations, leading to potential complications. Manufacturers might have to undertake additional measures to optimize performance and reliability.
Physical Properties of Semiconductors
Semiconductors are vital materials in the electronics industry, and understanding their physical properties is essential. These properties govern how semiconductors function within various applications. A semiconductor is characterized primarily by its electrical conductivity, which lies between that of conductors and insulators. Conductivity isn't constant; it varies with temperature, impurities, and other external factors. These variations provide vast possibilities for technological developments.
Key Physical Properties
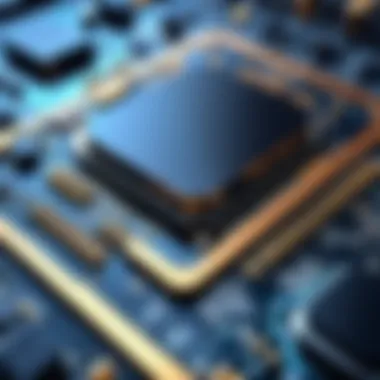
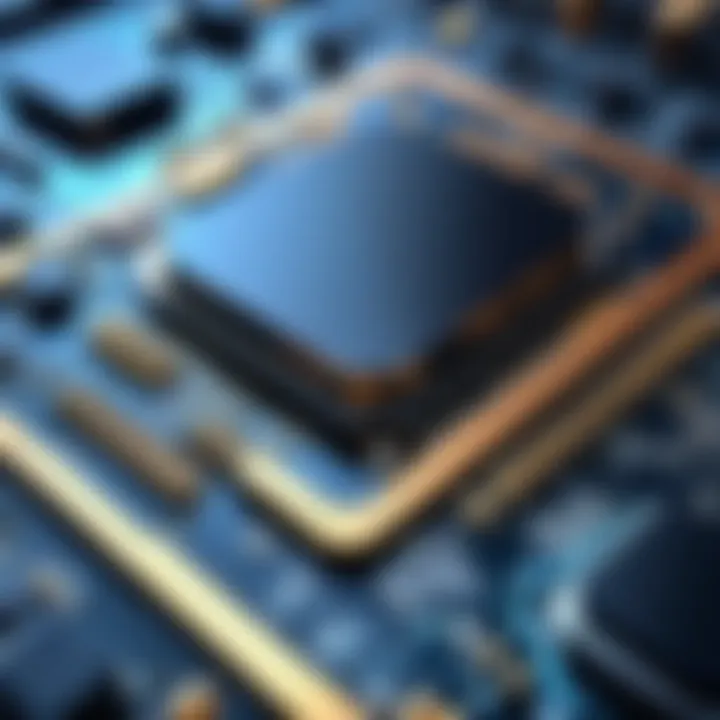
Here are some significant physical properties of semiconductors:
- Electrical Conductivity: This property seems simple, but it forms the core of semiconductor functionality. Such materials offer increased conductivity when temperature rises. This behavior is contrary to metallic conductors ones, emphasizing the unique nature of semiconductors.
- Band Gap Energy: Each type of semiconductor has an energy gap that separates the valence band from the conduction band. This band gap determines which wavelengths of light the material can absorb and thus influences the semiconductor’s efficiency in devices like photovoltaic panels.
- Temperature Dependence: The electrical properties vary with temperature changes. Generally, as temperatures go up, excitation occurs, allowing more electrons to jump the band gap, resulting in heightened conductivity.
The interplay between temperature and conductivity defines the performance of semiconductor devices.
Importance of These Properties
- Semiconductors are crucial in microelectronics, and understanding band gaps helps predict materials for specific applications.
- The knowledge of doping levels allows for tuning electrical characteristics, vital in designing various devices like transistors and diodes.
Considerations
When designing devices, engineers must consider the following factors:
- High-Temperature Operation: Semiconductor devices may experience challenges at elevated temperatures, leading to performance shifts. A landscape upon which reliability is tested.
- Defects and Irregularities: Presence of defects can drastically affect performance. Engineers must focus on material purity and device architecture to minimize these impacts.
In essence, understanding these physical properties allows for the optimization of existing technologies and the development of future innovations. It lays the foundation for advancements in the semiconductor industry and helps professionals make informed decisions regarding the materials and technologies that will define modern electronics.
Electronics and Band Theory
Electronics and band theory form a fundamental principle in understanding the behavior of semiconductors. This aspect addresses how electrons move within a material and how these movements contribute to electrical conduction. In essence, it establishes a framework for comprehending the various types of semiconductors outlined previously. Grasping this concept is crucial for anyone involved in semiconductor applications, from designers to engineers.
Importance of Band Theory
Band theory aids in explaining the electrical properties of materials, particularly semiconductors. In a semiconductor, energy bands separate the electrons' allowed states from their forbidden states. The two key bands include the valence band, where electrons are usually found, and the conduction band, where they can move freely and contribute to conductivity. The gap between these bands, known as the band gap, changes fundamental properties, making it essential to both functional applications and characteristics. Devices can operate effectively if designed with appropriate materials within these band contexts.
Key Concepts in Band Theory
Some central concepts in band theory include:
- Conduction Band: A higher energy level where electrons can travel without hindrance.
- Valence Band: The energy level occupied by atoms' electrons, critical to bond formation.
- Band Gap: The energy difference between the conduction band and the valence band. This gap determines a material's conductive capabilities.
Different types of semiconductors, such as silicon or gallium arsenide, exhibit distinctive band structures, affecting their uses in specific electronic components like diodes or transistors. Devices that rely more heavily on electron movement thrive in materials with narrower band gaps.
Applications Related to Electronics and Band Theory
Understanding electronics in conjunction with band theory opens doors to numerous applications:
- Diodes: Operate by controlling the flow of electrons through the conduction band, leading to light or alternative signals.
- Transistors: Utilize band theory to amplify or switch electronic signals, fundamental in computing technology.
- Solar Cells: Exploit the properties of semiconductors to convert sunlight into electrical energy.
Band theory is an indispensable tool to unlock the secrets of semiconductor technology, shaping innovations across diverse tech sectors.
Culmination
In summary, electronics and band theory inseparably link the nature of semiconductors to their functions. It serves as a keystone to developing advanced technology, allowing users a deeper appreciation for how mobility and electrical conduction determine efficiency and performance. A firm grasp of these concepts greatly enhances understanding in both academic and practical contexts among those involved with semiconductors.
Doping Processes in Semiconductors
Doping is a critical process in the fabrication of semiconductors. It involves adding impurities to an intrinsic semiconductor material to enhance its electrical conductivity. The primary goal of doping is to modify the electrical properties of the semiconductor, making it suitable for various applications in electronic devices. Understanding how doping works can elucidate its significance in the broader context of semiconductor technology.
The addition of dopant materials allows controlled alterations in charge carrier concentrations. This adjustment enables materials to transition between insulating and conductive states, essential for constructing transistors, diodes, and other electronic components. The implications of doping extend to performance enhancement, efficiency improvement, and functionality in devices. When done correctly, this process can significantly upgrade the semiconductor's suitability in applications ranging from consumer electronics to renewable energy solutions.
N-type Doping
N-type doping introduces impurities that have more valence electrons than the semiconductor material, predominantly silicon. Common shapeshifting dopants include phosphorus and arsenic. This introduces extra free electrons that can be harnessed for electrical conduction.
Benefits of N-type Doping:
- Increases charge carrier concentration, enhancing conductivity.
- Composes the electron donor type of semiconductor, conducive in rectifier and amplifier devices.
- Facilitates the creation of p-n junctions required for diodes and transistors.
N-type doped materials display distinct electrical characteristics that advantage them in various roles within the electronics sector, mainly where faster charge movement is beneficial. Devices compensating with N-type materials often conduct better when subjected to electric fields, thus increasing performance efficiency and responsiveness in application contexts.
P-type Doping
Conversely, P-type doping involves adding acceptor impurities that have fewer valence electrons than the semiconductor. Boron and gallium are among the usual candidates for such doping. The process creates ‘holes’ or places where electrons could fit into the crystalline structure, providing positive charge carriers for conduction.
Benefits of P-type Doping:
- Enhances mobility of holes, leading to efficient charge transport.
- Functions effectively in establishing the p-n junction, crucial for effective semiconductor devices.
- Permits interactions necessary for more complex configurations in bi-polar junction transistors.
Through P-type doping, semiconductor materials gain unique conductive properties that form the basis of many modern electronic devices. A fair blend of both N-type and P-type semiconductors facilitates the creation of essential components that underlie smart electronics, telecommunications, and advances in renewable energy technologies.
Applications of Semiconductors
Semiconductors are central to the advancement of technology across multiple industries. Their ability to control electrical current underlies many electronic devices and systems we use today. Understanding the applications of semiconductors helps in appreciating their significance in widespread technologies. Each type of semiconductor plays a distinct role in various sectors, driving innovation, optimizing performance, and lowering costs.
Consumer Electronics
Semiconductors are fundamental to consumer electronics, which include smartphones, tablets, and home appliances. These devices leverage semiconductor technologies for functions like processing, sensing, and data acquisition. Silicon, as an elemental semiconductor, forms the basis of many integrated circuits.
Here are notable applications in consumer electronics:
- Smartphones: Integrated circuits enable high-speed computation, allowing users to run multiple applications simultaneously.
- Televisions: Display technologies depend on compound semiconductors like Gallium Arsenide for improved picture quality.
- Smart Home Devices: Systems utilize sensor chips to manage energy consumption, enhancing efficiency.
The combination of convenience and functionality facilitated by semiconductors has made consumer electronics indispensable in our daily lives.
Computing Devices
Computing devices are another major area utilizing semiconductors effectively. Laptops, desktops, and servers rely heavily on both intrinsic and extrinsic semiconductors for optimal performance. They conduct and switch electrical signals at remarkable speeds, enabling devices to execute complex algorithms and run advanced software.
In computing, important areas of focus include:
- Microprocessors: Composed mainly of silicon, they act as the brain of computing devices, determining processing power and capabilities.
- Graphics Processing Units (GPUs): These units utilize specialized semiconductor materials to render images and visuals in response to demanding applications.
- Memory Storage: Solid-state drives store data using semiconductor technology, offering significant performance advantages over traditional hard drives.
The implications are vast since high-performance computing underpins many aspects of modern work and life, from fields such as data science to artificial intelligence.
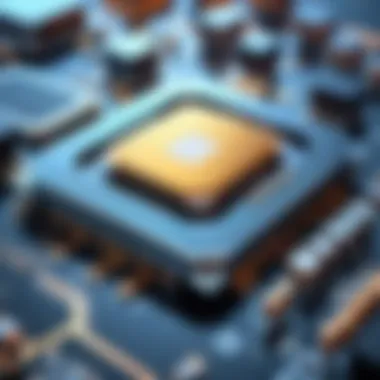
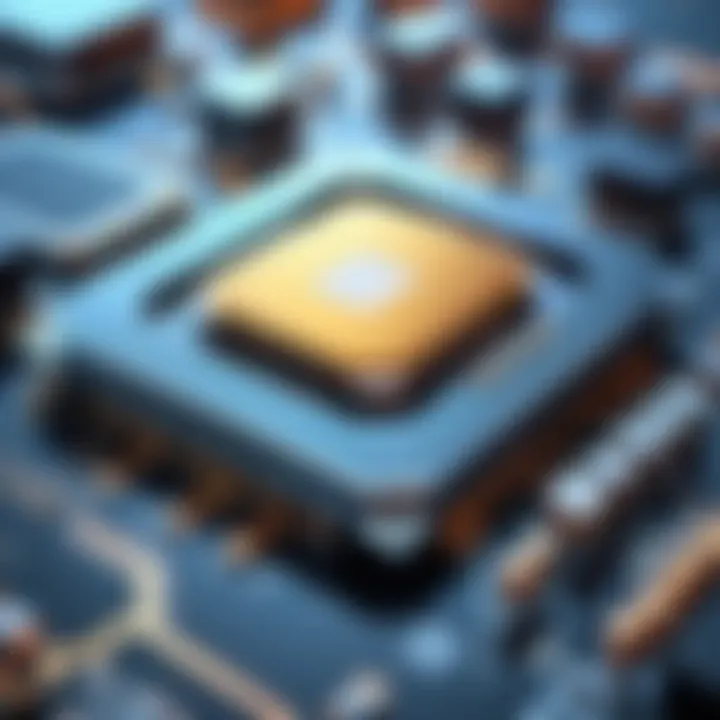
Telecommunications
Telecommunications significantly benefits from advanced semiconductor technologies. The demand for faster and more reliable connections drives improvements in semiconductor materials and devices.
Key applications in telecommunications include:
- Network Infrastructure: Devices like routers and switches rely on high-performance semiconductors to maintain steady data throughput.
- 5G Technology: Compound semiconductors enable the high-frequency operations necessary for 5G networks, providing improved bandwidth and reduced latency.
- Satellites: Semiconductor technology plays a role in enabling accurate and stable communication systems used in satellite technology.
The evolution and enhancement of telecommunications directly depend on innovations in semiconductor applications.
Renewable Energy Solutions
The role of semiconductors in renewable energy is increasingly vital as the world moves towards sustainable solutions. Technologies such as solar power and energy storage systems rely on semiconductors to optimize energy use.
Some notable applications are:
- Photovoltaic Cells: These cells utilize intrinsic and compound semiconductors to convert sunlight into usable electricity efficiently.
- Inverters: They enable energy to be transferred to power grids, where semiconductors ensure the desired voltage and frequency.
- Smart Grids: Multiple semiconductor-based devices collect data to balance energy loads across the distribution network more effectively.
As technology advances, the integration of semiconductors into renewable energy solutions will be critical in enhancing growth and sustainability in the energy sector.
The versatility of semiconductors enables transformative changes across multiple domains, making fundamental contributions to technological advancements.
In summation, semiconductors are woven into the fabric of modern technology. The applications discussed confirm their critical role in driving innovation across various fields. As we continue to advance, the importance of these materials will only grow further.
Future Trends in Semiconductor Technology
As we look ahead, the semiconductor industry is undergoing rapid transformation. Understanding the future trends in semiconductor technology is crucial. This section explores key developments that will reshape how semiconductors are designed, manufactured, and deployed in various applications.
One trend is the miniaturization of components. Smaller transistors improve performance while lowering power consumption. In this frame, 3nm process technology is emerging as a game changer, promising considerable gains in power efficiency. Companies like TSMC and Intel are leading this move to create smaller, faster chips.
Parameters such as size, efficiency, and manufacturing costs become increasingly important. This shift can significantly impact different sectors including computing and consumer electronics.
Focus on Materials
Another significant shift is the exploration of new materials. Traditional silicon is still predominant, but gallium nitride and silicon carbide are being adopted for particular high-performance and high-frequency applications. These materials exhibit superior electric properties, enabling devices to perform under strenuous conditions, which benefits renewable energy systems and electric vehicles.
- Gallium Nitride: Useful for RF applications.
- Silicon Carbide: Beneficial for high-voltage components.
Furthermore, the increasing adoption of quantum computing introduces next-generation semiconductors that rely on quantum bits or qubits rather than classical bits. Efficient qubit development requires specialized semiconductor designs and structures.
Artificial Intelligence and Machine Learning
Semiconductors will also play a pivotal role in the growth of artificial intelligence (AI). The demand for powerful computation to enable AI is surging.
- Neuromorphic chips mimic brain functions, enhancing machine learning.
- Tensor Processing Units (TPUs) specifically optimize AI workloads, boosting the speed and efficiency of neural network processing.
Global Supply Chain Considerations
In light of current global events, supply chain resilience becomes fundamental. Geopolitical factors and natural disasters can disrupt production, affecting the availability and price of semiconductors. As such, industries are revisiting supply chains to ensure stable access to resources.
Challenges in Semiconductor Development
The field of semiconductor development is complex, marked by continuous innovation and growth. Numerous challenges arise, influencing the pace of advancements and their applicability in various sectors. Understanding these challenges is essential for appreciating the broader semiconductor context.
One primary challenge is the material limitations in semiconductor manufacturing. While materials like silicon dominate the landscape, the physical properties of materials dictate the efficiency and performance of devices. Transitioning to new materials or compounds, such as graphene or gallium nitride, presents cost and scalability issues that must be addressed. Operational behaviors, such as defect densities and bandgap characteristics, thus must be carefully studied.
Economic factors also play a pivotal role. The research and development costs can be exorbitant. Developing new semiconductors often requires finite resources, impacting smaller entities without vast funding. As markets fluctuate, maintaining balance in investment can lead to unsure long-term ROI, making financing R&D efforts complicated.
A crucial aspect to consider involves global supply chain dynamics. The recent turmoil, exacerbated by geopolitical tensions and public health crises, has spotlighted vulnerabilities in supply chains. Material shortages have resulted in significant impacts on production capabilities and timelines. Strains in connectivity further impact the flow of materials and finished products.
Regulatory standards and compatibility also pose hurdles. Adhering to stringent environmental and safety regulations complicates processes. For instance, California’s Proposition 65 and Europe’s Union REACH regulations impose strict definitions on semiconductor materials, affecting development timelines and practices. This results in increasing compliance costs and potential pushes for alternative safer materials.
Green energy discourse necessitates change within semiconductor development as well. With increasing sustainability concerns, developers are bound to find greener production methods or waste management techniques. The future is leaning toward eco-conscious production practices, demanding greater efficiencies without compromising performance.
"Understanding challenges in semiconductor development allows for strategic innovations that harness greater efficiency and outreach in numerous applications."
Addressing these challenges not only empowers the semiconductor field but also promotes resilience against future adversities.
The Role of Semiconductors in Artificial Intelligence
Semiconductors are fundamental in the development and functionality of artificial intelligence (AI). They are crucial for processing large amounts of data and performing complex computations necessary for AI applications. The characteristics of semiconductors, such as their ability to conduct electricity or act as insulators, make them pivotal in various AI systems.
One main benefit of semiconductors is their capacity for miniaturization. This trait enables the creation of smaller devices with significant processing power, essential for AI. Moreover, the increasing efficiency of semiconductors correlates directly with elevating the capabilities of AI systems, driving advancements in machine learning and neural networks. For example, graphic processing units (GPUs) are primarily built on semiconductor technology, facilitating rapid data processing for tasks like image recognition, deep learning, and natural language processing.
Several considerations emerge when discussing the role of semiconductors in AI:
- Performance: Look at specific semiconductor types high-performance applications must support. For instance, silicon-based semiconductors traditionally form the backbone, but alternatives like gallium nitride and silicon carbide show increasing promise.
- Energy Efficiency: As AI computations consume substantial power, energy-efficient semiconductors lower operating costs and heat generation, becoming a significant consideration in AI deployment.
- Scalability: The ability to scale up semiconductor production to meet rising demand for AI devices under pins the industry's growth.
In essence, the integration of semiconductors propels AI technologies forward, enhancing their capabilities while providing the foundation on which advanced systems operate. Consequently, an awareness of the semiconductor space reinforces a deeper understanding of the complexities and necessities guiding AI's evolution.
The advancements in semiconductor technology will determine how effective artificial intelligence becomes across various industries.
The relationship between semiconductors and artificial intelligence will likely continue to evolve. As new materials and fabrication techniques emerge, the capabilities and applications will expand further. This progression presents numerous opportunities and challenges, shaping the future landscape of AI and its interaction with semiconductor technologies.
Culmination
In examaning the types of semiconductors and their numerous applications, it becomes increasingly clear that semiconductors are at the very heart of modern technology. Their unique conductive properties, stemming from their intrinsic and extrinsic nature, allow for tailored functionality in a vast array of devices. This diversity is imperatice in areas from simple consumer electronics to more sophisticated applications in computing and telecommunications.
Importance of Semiconductors
Semiconductors, such as silicon and gallium arsenide, are fundamental to the construction of transistors, diodes, and integrated circuits. These components are critical for the functionality of virtually all electronic realizaions today. Recognizing their role not only enhances the understanding of electronic devices but also highlights how advances in semiconductor technology can drive future innovations.
The significs of semiconductors extends beyond basic functionality. They play a pivotal role in facilitating energy-efficient solutions and groundbreaking advancements in artificial intelligence. Their instrumental role in logics circuits allows performance improvisation while conserving energy—a key consideration in our increasingly power-conscious world.
"Semiconductors are not just building blocks; they are conduits of innovation facing modern challenges."
Future Trends
Looking forward, several trends suggest increased complexity in tasks lead by future semiconductor technology. Notably, quantum computing independs on the breakthrough development of multi-state semiconductors. Investments in research, particularly around compound ore gaelic semiconductors, hint at potential industry expanstion. Moreover, as the world aims for sustainability, semiconductors will undoubtly have a large presence in renewable energy tech.
Epilogue Reflections
To summarize, understanding types of semiconductors is important for aspiring electronics and technology professionals. Knowledge of their unique properties, efficiencies, and application areas enables better grasping their implications for societal needs and continuing transformation in tech landscape. For anyone stepping into this field, continuous learning about emerging semiconductor developments would be invaluable. Their applications not only facilitate advancements but also remain essential in adapting to windows of opportunity within the tech sphere.