Mastering Operational Amplifiers: A Comprehensive Guide
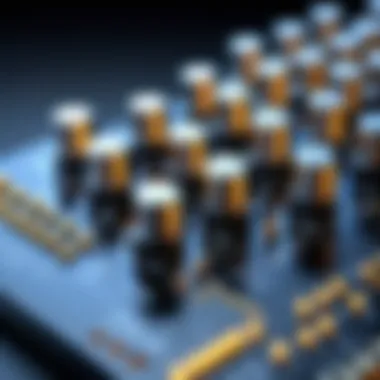
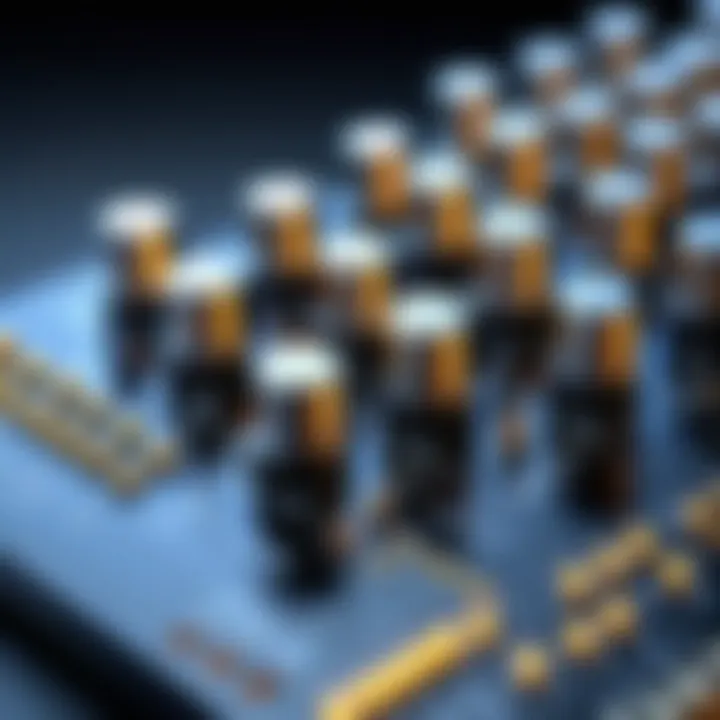
Overview of Topic
Operational amplifiers, often called op-amps, serve as one of the fundamental components in electronic circuits. Their versatility extends to various applications ranging from signal amplification to complex mathematical operations. Op-amps are crucial in analog electronics and play a significant role in modern technology.
Historically, the operational amplifier came into existence in the late 1960s. They were initially designed for specific mathematical operations, such as addition, subtraction, integration, and differentiation. The evolution of op-amps can be traced back to their rudimentary forms in vacuum tubes, transitioning into today's integrated circuits, which exemplify advancements in engineering and technology.
In this article, we will explore the structure and functionality of operational amplifiers. Emphasis will be placed on their operational principles as well as configurations that make them adaptable for various functions. Understanding these devices is vital for those pursuing careers in electronics, engineering, or any field that involves circuit design.
Fundamentals Explained
Key terms related to op-amps include:
- Gain: The ratio of output voltage to input voltage, which signifies how much an op-amp amplifies a signal.
- Negative Feedback: A method to stabilize gain and control output by feeding a portion of the output back to the input.
- Offset Voltage: The voltage that must be applied to the input terminals to make the output zero.
The foundational knowledge also encompasses understanding various configurations such as inverting, non-inverting, and differential amplifiers. Each configuration possesses unique properties that cater to different application needs.
Practical Applications and Examples
Op-amps find their use in a wide array of real-world applications. Some notable examples include:
- Audio amplifiers, which enhance sound signals for better quality.
- Signal conditioning, used to adjust signals for processing and analysis.
- Analog calculators that perform mathematical operations on analog inputs.
Hands-on projects can involve building simple amplifiers or integrating op-amps into larger systems. For practical implementation, resources like data sheets from manufacturers often provide insight into circuit diagrams and specifications.
For example, in implementing a non-inverting amplifier, one could use a simple resistor configuration that follows:
where V_out is the output voltage, V_in is input voltage, R_f is feedback resistor, and R_i is input resistor.
Advanced Topics and Latest Trends
As the field of electronics advances, operational amplifiers have also seen significant development. Current trends include the integration of op-amps with digital circuits, known as mixed-signal processing. Researchers are also exploring low-power op-amps suitable for battery-operated devices.
Additionally, innovations in materials and circuit design lead to improved performance characteristics. These developments paved the way for high-frequency applications and enhanced precision in measurements.
Future prospects indicate a growing relevance in areas like sensor integration, medical devices, and communication technology.
Tips and Resources for Further Learning
For those keen on expanding their knowledge about operational amplifiers, several resources are available:
- Books: "Op-Amps for Everyone" by Ron Mancini provides comprehensive coverage of op-amps and their applications.
- Online Courses: Platforms like Coursera or edX offer courses focused on electronics and circuit design, focusing on op-amps.
- Tools: Software such as LTspice for circuit simulation can aid in understanding op-amp behavior in various configurations.
Continuous learning and hands-on practice will enhance expertise in using operational amplifiers effectively in different endeavors.
Intro to Operational Amplifiers
Operational amplifiers, often referred to as op-amps, are crucial components in the field of electronics. They are designed to amplify voltage signals and can perform a variety of functions in circuits. This section serves as an entry point into understanding these versatile devices.
Importance of Operational Amplifiers
Operational amplifiers have revolutionized signal processing due to their high voltage gain and flexibility. They can be configured in numerous ways, making them essential for tasks such as filtering, integration, and even comparison of signals. Understanding the core ideas behind op-amps allows engineers and technicians to leverage these devices effectively in various applications. As the foundation for numerous electronic systems, knowledge about op-amps opens the door to mastering more complex electronic concepts.
Benefits of Studying Operational Amplifiers
- Versatility: Op-amps can be used in different configurations, such as inverting and non-inverting setups, enhancing their functional utility in various circuits.
- Performance: Their ability to boost weak signals makes them indispensable in signal conditioning applications.
- Accessibility: Resources for learning about op-amps are widely available, making it easier for novices and professionals alike to explore their potential.
Key Considerations
When learning about operational amplifiers, it is essential to consider their practical aspects as well as their theoretical basis. Understanding the limitations, such as bandwidth and power supply requirements, helps in designing effective circuits. Additionally, grasping the fundamental concepts behind feedback mechanisms can significantly improve circuit performances.
"Operational amplifiers are a cornerstone of modern electronics, integrating effortlessly into a multitude of applications, showcasing their fundamental importance."
In summary, operational amplifiers are not merely electronic components; they are the backbone of many circuits in today's technology-driven world. The insights gained from this article will provide the reader with a solid foundation for applying op-amps in practical scenarios.
Basic Structure of Operational Amplifiers
Understanding the basic structure of operational amplifiers (op-amps) is crucial for grasping their functionality and versatility in electronic applications. The structural design influences the performance characteristics of these devices, making this section essential for students and professionals alike. By examining both the components that form an op-amp and their schematic representation, we can appreciate how these elements interact to achieve desired outcomes in circuit design.
Components of an Op-Amp
An operational amplifier is composed of several key components, each serving a unique function. The main components include:
- Differential Inputs: Most op-amps have two input terminals: the inverting (-) and the non-inverting (+) input. These inputs receive the input signals and their difference is amplified.
- Output: The output terminal produces the amplified signal. It reflects the weighted difference of the input signals.
- Power Supply Terminals: Op-amps require a dual power supply, usually represented as V+ and V-. These terminals supply the necessary voltage for operation.
- Internal Transistors: Op-amps contain a series of transistors, typically bipolar junction or Field Effect Transistors, configuring them for gain and other functions.
- Resistors and Capacitors: Additional passive components within the op-amp play crucial roles in stability and bandwidth.
Every component is meticulously designed and selected to optimize performance, minimize noise, and ensure linear amplification. Their synergy is what allows op-amps to fulfill diverse roles in signal processing.
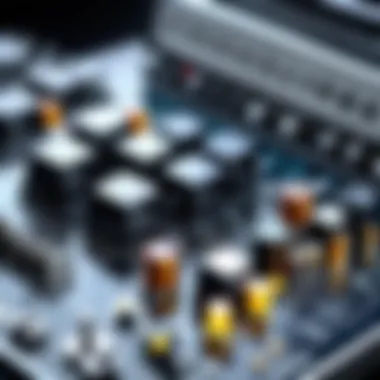
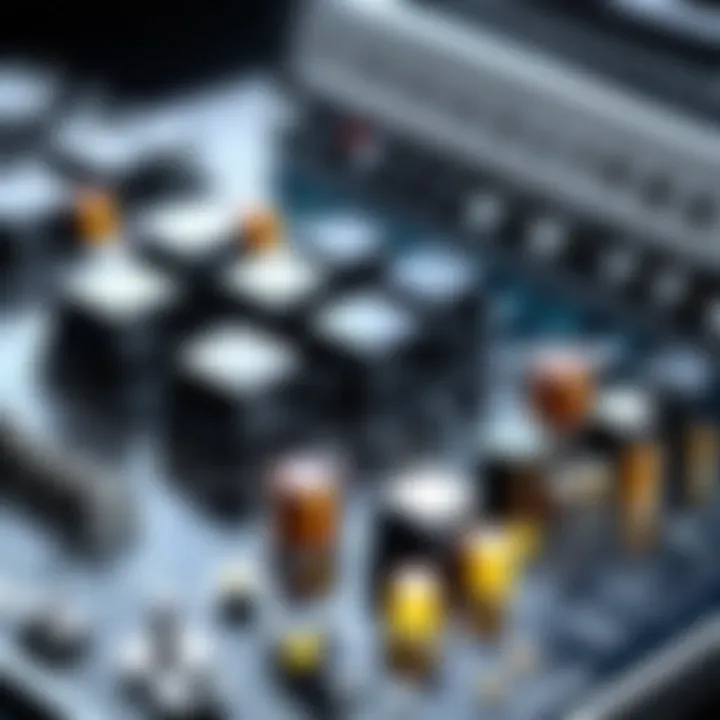
Schematic Representation
The schematic representation of an op-amp simplifies the understanding of its structure and operation. Usually depicted as a triangle, the schematic consists of the following essential features:
- Symbol Anatomy: The triangle symbol prominently displays two input terminals and one output terminal. The orientation of the terminals indicates the flow of signals.
- V+ and V- Labels: These labels mark the power supply connections, indicating where the positive and negative voltages are sourced from.
- Input and Output Arrows: Arrows indicate the direction of current flow, establishing clear relationships between inputs and output.
The schematic diagram provides an abstract view while retaining technical details essential for design and analysis.
For circuit designers, itβs crucial to interpret these schematics accurately. A clear understanding can lead to informed decisions when developing circuits that utilize op-amps. Whether designing a filter, an amplifier, or integrating with microcontrollers, recognizing the structural fundamentals of op-amps significantly impacts operational success.
In summary, the basic structure of operational amplifiers, comprising their core components and schematic representation, fundamentally shapes their function in various applications. Understanding these elements lays the groundwork for deeper insights into operational principles and configurations of op-amps that will follow in the article.
Operational Principles
The operational principles of operational amplifiers (op-amps) are fundamental to grasping their functionality and applications in electronic circuits. Understanding these principles is crucial for anyone looking to manipulate and design circuits effectively. When diving into the operational principles, one must consider how op-amps process input signals, the role of feedback, and the resultant behavior of the amplifier.
Inverting and Non-Inverting Inputs
Op-amps function with both inverting and non-inverting inputs, which are essential for determining output behavior. The inverting input works by applying the input signal to this terminal. The output will be voltage inverted regarding this input. It serves to amplify signals while flipping their phase. For example, if a positive voltage is applied to the inverting input, the output will reflect a negative voltage, thus providing a gain that flips the signal.
In contrast, the non-inverting input allows the input signal to be processed without an inverted output. When the input is applied here, the output mirrors the phase of the input. This type of input is useful in applications where preserving the input signal's phase is essential. The gain for a non-inverting configuration can be defined with the equation:
where ( R_f ) is the feedback resistor and ( R_in ) is the resistor connected to the ground. Understanding this distinction enhances one's ability to design and analyze circuits precisely as intended.
Feedback Mechanisms
Feedback mechanisms are vital in the operation of op-amps. Feedback refers to the portion of the output that is returned to the input. It influences the overall gain, stability, and linearity of the amplifier. There are two primary types of feedback: positive and negative.
- Negative feedback is crucial in most op-amp applications. It decreases the gain but increases stability. This form of feedback ensures that the op-amp operates within its linear range, minimizing distortion and enhancing accuracy. Moreover, negative feedback allows for controlling the gain precisely, enabling predictable outcomes in circuit design.
- Positive feedback, on the other hand, could lead to instability. When the output is fed back in phase with the input, it boosts gain to a point where it can push the op-amp into saturation. Saturation is a state where the output cannot increase any further regardless of the input. Although this can be useful in certain applications, such as comparators or oscillators, it is typically avoided in linear applications.
The relationship between feedback and output can be summarized in the statement:
"Negative feedback stabilizes and perfects amplifier performance, while positive feedback drives it to extremes."
Common Configurations of Op-Amps
Understanding the common configurations of operational amplifiers (op-amps) is essential in grasping their applications in electronic circuits. These configurations define how op-amps are utilized to achieve specific functions. By effectively configuring op-amps, designers can optimize circuit performance, improve signal integrity, and ensure desired outcomes. The four primary configurations are the inverting amplifier, non-inverting amplifier, voltage follower, and differential amplifier. Each comes with its unique characteristics, benefits, and considerations that are crucial in various scenarios.
Inverting Amplifier Configuration
The inverting amplifier configuration utilizes the inverting input of the op-amp to produce an output that is both amplified and inverted in phase. This means that if the input signal is positive, the output signal will be negative, and vice versa. The gain of the inverting amplifier is determined by the ratio of two resistors: the input resistor (R1) and the feedback resistor (R2).
The formula to calculate gain (A) is:
[ A = -\fracR2R1 ]
Key advantages of the inverting amplifier include:
- Signal Phase Inversion: The output is inverted, which can be useful in circuits requiring precise phase control.
- Wide Range of Gain: By altering resistor values, gain can be adjusted easily according to needs.
- Impedance Matching: It provides a low input impedance, making it suitable for applications involving high-frequency signals.
However, it does have limitations, such as the difficulty in achieving a high input impedance unless a buffer stage is added.
Non-Inverting Amplifier Configuration
In contrast, the non-inverting amplifier configuration allows the input signal to be fed directly into the non-inverting input terminal. The output signal is in phase with the input. Similar to the inverting amplifier, the gain is still determined by resistor values.
The gain can be expressed as:
[ A = 1 + \fracR2R1 ]
Some noteworthy benefits include:
- Phase Preservation: The output maintains the same phase as the input, essential for applications where phase alignment is critical.
- Higher Input Impedance: This configuration boasts a high input impedance, minimizing the loading effect on the previous stage.
- Easier to Design: Simplicity in calculations and designs is often more manageable than the inverting configuration.
Yet, it does require careful attention to feedback resistors to maintain stability in high-gain applications.
Voltage Follower Configuration
The voltage follower, also known as a unity gain buffer, is a special case of the non-inverting amplifier where the gain is precisely one. This configuration allows the op-amp to output the same voltage level as the input while providing significant current gain.
The voltage follower is beneficial for:
- Impedance Buffering: It decouples the source from the load, preventing any loading effect on the preceding circuit.
- Signal Isolation: Acting as an isolation stage can protect sensitive source circuits from heavy loading.
Overall, this configuration is simple yet powerful, often used when signal integrity must be maintained without amplification.
Differential Amplifier Configuration
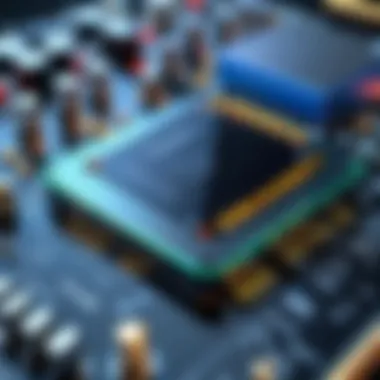
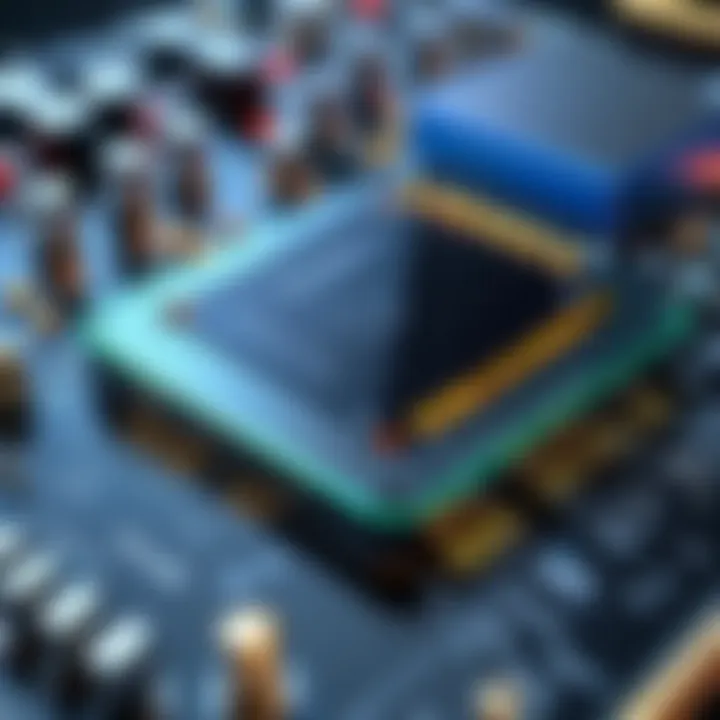
The differential amplifier configuration showcases the unique capability of op-amps to amplify the difference between two input voltages. It utilizes both inverting and non-inverting inputs, offering excellent robustness to common-mode noise.
The basic expression for the differential amplifier's output is:
[ V_out = A(V_2 - V_1) ]
Where V1 and V2 are the input voltages. The benefits of this configuration include:
- Noise Rejection: It effectively cancels out noise since it amplifies only the difference between inputs.
- Versatile Applications: It is widely used in sensor and signal processing applications where differential signaling is required.
Applications of Operational Amplifiers
Operational amplifiers are versatile components found in numerous electronic devices and systems. Their utility spans across various fields, such as audio processing, automation, and instrumentation. Understanding the unique applications of op-amps not only highlights their significance in electronics but also allows for practical insight into their implementation. The benefits of utilizing op-amps include enhanced circuit performance, flexibility in design, and precise control over signals. Below are several primary applications that showcase the capabilities of operational amplifiers in real-world scenarios.
Signal Conditioning
Signal conditioning is a crucial application of operational amplifiers. This process involves manipulating a signal to make it more suitable for processing. By adjusting parameters like amplitude and impedance, op-amps can greatly improve signal quality. For instance, sensors often produce weak signals that need to be amplified before further processing.
- Benefits of signal conditioning with op-amps:
- Increased signal strength and clarity
- Removal of noise and unwanted frequencies
- Conversion between signal types (e.g., analog to digital)
Through signal conditioning, operational amplifiers ensure that system inputs meet specific requirements, enhancing overall system performance.
Filters and Integrators
Filters and integrators are fundamental in processing signals, and operational amplifiers play a vital role in their design. Active filters utilize op-amps to selectively attenuate or amplify certain frequency ranges in a signal. This capability is essential in applications such as audio equipment where specific frequencies may need enhancement or reduction.
- Types of filters include:
- Low-pass filters
- High-pass filters
- Band-pass filters
- Notch filters
Integrators, on the other hand, accumulate input signals over time, producing an output proportional to the integral of the input voltage. This is particularly useful in applications requiring precise timing or signals like in analog computers.
Comparators
Operational amplifiers can function as comparators, which are used to compare two input voltages and determine which is higher. This application is widespread in control systems and level detection circuits. In this role, an op-amp outputs a high or low signal based on the comparison of the inputs, allowing for straightforward decision making within circuits.
- Applications of comparators include:
- Zero-crossing detection
- Comparator circuits in analog-to-digital converters
- Voltage level monitoring
Using op-amps as comparators enhances system responsiveness and precision in operations needing rapid comparisons.
Oscillators
Oscillator circuits, which generate periodic waveforms, often utilize operational amplifiers to create sine, square, or triangular waves. These circuits are integral to various applications ranging from clock generation in digital systems to signal generators in testing equipment. Op-amps provide a stable and adjustable means to generate these waveforms.
- Key aspects of op-amp oscillators include:
- Simple design configurations
- Stability across varying frequencies
- Adjustable output frequency and amplitude
The ability of operational amplifiers to produce accurate oscillations makes them valuable in numerous electronic applications.
Instrumentation Amplifiers
Instrumentation amplifiers, a specialized type of op-amp, are essential in applications requiring high accuracy and high input impedance. They are particularly suitable for amplifying low-level signals from sensors in medical devices and industrial applications. Their design features allow for excellent noise rejection and minimal drift, which are critical in maintaining signal integrity.
- Characteristics of instrumentation amplifiers:
- High common-mode rejection ratio (CMRR)
- Ability to handle differential signals
- Versatile application range, from medical instrumentation to data acquisition systems
Performance Specifications
Performance specifications of operational amplifiers are critical because they determine how well the device will perform in various applications. Understanding these specifications allows engineers and technicians to select the right op-amp for a specific task, ensuring optimal performance in electronic circuits. Each specification provides insights into the capability of the op-amp in relation to the signal it is processing.
In this section, we will focus on four key performance specifications: gain and frequency response, input and output impedance, common mode rejection ratio (CMRR), and power supply rejection ratio (PSRR). These factors can significantly impact the reliability and efficiency of circuits that utilize operational amplifiers.
Gain and Frequency Response
The gain of an operational amplifier is a measure of how much an input signal is amplified at the output. It is typically specified in a ratio or decibels (dB). A high gain indicates that small input signals can produce large output signals, which is often desirable in many applications.
Frequency response describes how the gain changes with frequency. Op-amps are not ideal and can show reduced gain at higher frequencies, which is important to consider in applications like audio processing or signal filtering. Understanding the frequency response can help users predict how the op-amp will behave under different conditions.
Input and Output Impedance
Input impedance refers to the resistance of the op-amp to incoming signals. A high input impedance is preferred, especially for applications dealing with sensitive signals, as it minimizes the load on the preceding circuit. An op-amp with low input impedance can load down the source and distort the signal.
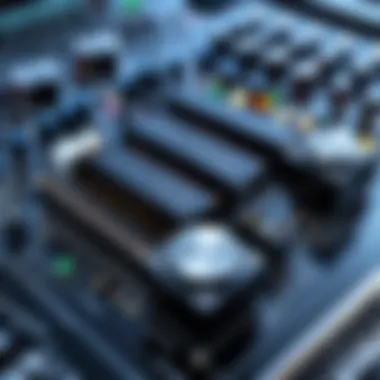
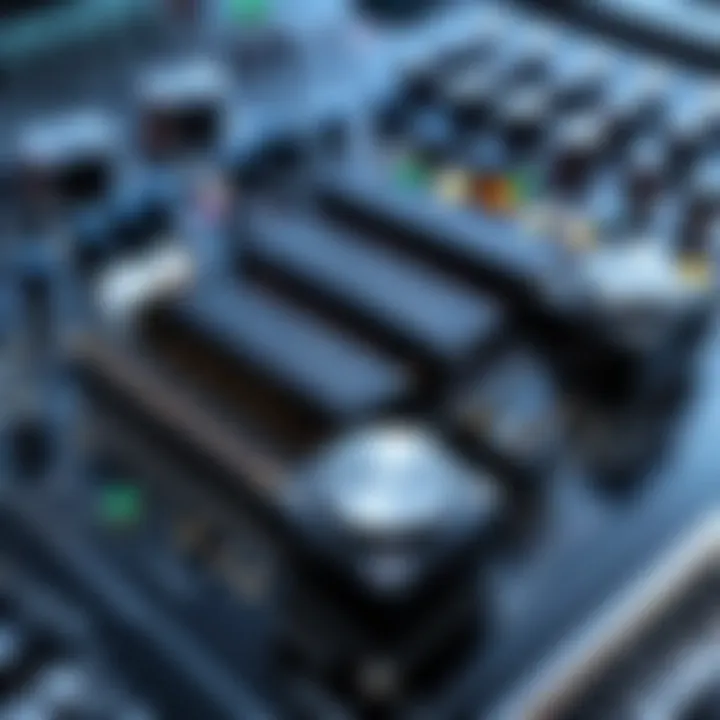
Output impedance, on the other hand, is the resistance at the output of the op-amp. It should be low to drive loads effectively without significant voltage drop across the output. The relationship between input and output impedance directly affects the overall performance and is vital for engineers to consider in circuit design.
Common Mode Rejection Ratio (CMRR)
CMRR is a crucial specification that quantifies an operational amplifier's ability to reject common-mode signals. These are signals that are present on both inputs simultaneously. A high CMRR indicates that the op-amp can effectively differentiate between a real differential input and noise or interference that affects both inputs. This is vital in applications like measuring small signals in the presence of noise, such as in instrumentation.
Power Supply Rejection Ratio (PSRR)
PSRR measures how well an op-amp can maintain its output performance despite variations in power supply voltage. This is important because fluctuations in the power supply can affect the operation of the amplifier and lead to inaccuracies in the output signal. A high PSRR indicates better stability and reliability in varying power conditions. Understanding PSRR is essential when designing circuits that are subjected to unstable power supply conditions.
"Choosing an operational amplifier involves more than just understanding basic operations; performance specifications are key to ensuring functionality in practical applications."
In summary, performance specifications are essential for anyone working with operational amplifiers. A thorough understanding of gain and frequency response, input and output impedance, CMRR, and PSRR allows users to make informed decisions in electronic design and application.
Advantages and Limitations
Understanding the advantages and limitations of operational amplifiers is crucial for anyone working in electronics. This knowledge provides insight into why they are favored in various applications and also highlights the constraints that might require alternative solutions. Each aspect serves to inform design choices, whether one's role is designing new circuits or troubleshooting existing systems. The focus on the advantages illustrates the versatility and performance of op-amps, while the limitations shed light on the need for careful integration and consideration of specific circuit requirements.
Advantages of Using Op-Amps
Operational amplifiers offer numerous advantages that make them essential components in modern electronics:
- High Gain: Op-amps provide substantial amplification, often exceeding 100,000 times, allowing for weak signals to be amplified significantly.
- Versatility: They can be configured in various ways to serve different functions, such as amplification, filtering, and comparisons. This adaptability increases their applicability in assorted circuits.
- Cost-Effective: Production and design of op-amps have become economical over the years. They are generally affordable, making them accessible for both DIY projects and industrial applications.
- Wide Frequency Range: Op-amps maintain stable performance over a broad frequency range, making them suitable for a variety of applications including audio and communications.
- Integration: Modern integrated circuit technology allows for multiple op-amps to be combined in a single package. This reduces circuit complexity and space requirements.
- Simplicity in Design: They simplify circuit designs. Even those with basic knowledge can employ op-amps effectively without intricate understanding of every aspect of signal processing.
These advantages underscore why op-amps are extensively used in everything from signal conditioning to instrumentation amplifiers, affirming their pivotal role in electronics.
Common Limitations
Despite their overall usefulness, operational amplifiers come with certain limitations that are essential to acknowledge:
- Voltage Range: The output voltage of an op-amp is limited by the supply voltage. This can restrict the application in circuits requiring higher voltages than the available power supplies.
- Slew Rate: Op-amps have a finite slew rate, which is the maximum rate at which the output can change. This can limit performance in high-frequency applications, where rapid changes in input signals occur.
- Input Bias Current: The small current that flows into the input terminals can introduce errors in high-impedance applications. Managing this bias current is vital to ensure signal integrity.
- Offset Voltage: There is often a small voltage difference between the input terminals even when ideal conditions are assumed. This offset can result in noticeable errors in precision applications, if not properly compensated.
- Common Mode Discrimination: While op-amps can reject common mode signals, they are not perfect at it. A high common mode signal can affect the differential response, potentially leading to inaccuracies.
Understanding these limitations allows users to make informed decisions when designing circuits or selecting alternative components to achieve desired outcomes.
Recognizing both advantages and limitations allows engineers and technicians to utilize op-amps effectively, yielding performance while managing risks associated with their weaknesses.
Future Developments in Op-Amps
The field of operational amplifiers is continually evolving. As technology advances, new designs and applications emerge to solve more complex problems. Future developments in op-amps are crucial for several reasons. First, they contribute to increasing the performance and efficiency of electronic devices. As demands for speed and accuracy rise in applications such as communication systems, the role of op-amps will become ever more significant.
Innovations in Op-Amp Design
Innovations in op-amp design focus on enhancing performance and reducing limitations. One notable advancement is the use of CMOS (Complementary Metal-Oxide-Semiconductor) technology. This design choice enables op-amps to achieve lower power consumption, which is especially valuable in battery-powered devices. Another trend is the integration of op-amps with digital circuits. This creates mixed-signal chips that combine analog and digital processing capabilities in one package, offering greater versatility and compactness.
Key innovations include:
- Low Power Consumption: Designs that consume less energy,
- Higher Gain Bandwidth Product: Improving frequency response,
- Enhanced Temperature Stability: Making them suitable for harsh environments,
- Built-in Calibration: Allowing for easier adjustment in practical applications.
These innovations not only push the boundaries of what operational amplifiers can do but also expand their usability in new applications.
Emerging Applications
As technology continues to develop, new applications for op-amps are emerging, broadening their relevance across industries. In the realm of healthcare, for instance, operational amplifiers are increasingly used in medical devices for signal conditioning. This allows for better data acquisition from sensors used in diagnostic equipment.
In the automotive sector, op-amps are now critical in various systems such as anti-lock braking systems (ABS) and electric power steering. They enhance the responsiveness and safety features of vehicles.
Moreover, the rise of the Internet of Things (IoT) has created a demand for smarter and more efficient devices. Op-amps play a vital role in sensor applications, where they help in processing analog signals from environmental sensors.
"Operational amplifiers represent a bridge between the analog and digital worlds, making them essential in modern technology."
Emerging applications include:
- Medical devices: Used for precision in diagnostics,
- Automotive systems: Enhancing control and safety,
- IoT devices: Processing signals from various sensors.
As we look to the future, it is clear that operational amplifiers will continue to shape the landscape of electronics through innovations in design and their application in various fields. Their adaptability ensures they remain relevant in an ever-changing technological world.
Culmination
Summary of Key Points
Operational amplifiers are versatile devices that can be configured in multiple ways to achieve desired amplification or signal processing. Key configurations include inverting and non-inverting amplifiers, voltage followers, and differential amplifiers. Each configuration has unique characteristics, making op-amps suitable for specific tasks in electronics.
Moreover, operational amplifiers are characterized by several performance specifications that must be considered in design. These include gain, frequency response, input and output impedance, common mode rejection ratio (CMRR), and power supply rejection ratio (PSRR). Each specification influences the op-amp's effectiveness and reliability in circuits.
The article also highlighted the advantages of using operational amplifiers, such as their high gain, versatility, and ease of use. However, it also discussed their limitations, including issues related to bandwidth and noise.
Importance to the Field of Electronics
Operational amplifiers play a pivotal role in the advancement of electronic systems. They are integral to numerous applications, from simple circuits to complex instrumentation. Their ability to process signals accurately and efficiently makes them essential in areas like signal conditioning, filtering, and control systems.
As technology evolves, the development of innovative op-amp designs and applications continues to grow. Emerging technologies, like those used in integrated circuits and advanced instrumentation, further underscore the importance of understanding op-amps in electronics.
"Operational amplifiers are the backbone of modern analog electronics, influencing a vast array of technologies and applications."
In summary, gaining proficiency in the use of operational amplifiers extends beyond theoretical knowledge. It opens doors to implementing real-world solutions, foster innovation, and promotes engineering excellence in the field of electronics.