Decoding the Components of Solar Cell Diagrams
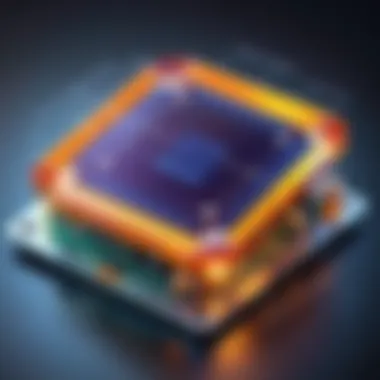
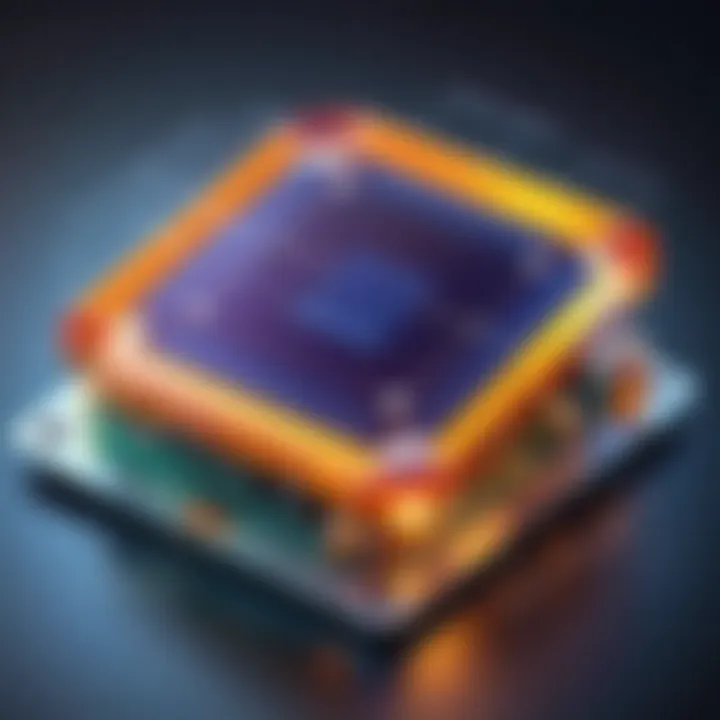
Overview of Topic
In a world increasingly driven by sustainable solutions, understanding the anatomy of solar cells becomes not just relevant, but essential. Solar cells, primarily used in photovoltaic systems, are pivotal in harnessing the sun’s energy, turning sunlight into usable electricity. This topic dives into the intricate diagram of solar cells, unraveling their components, functions, and significance in the broader tech landscape.
The scope of this article encompasses a thorough exploration of each segment within a solar cell diagram. We’ll touch upon:
- The Main Components: Identifying and explaining crucial parts of solar cells.
- Functionality and Operation: Understanding the interplay between components.
- Historical Evolution: How technology has progressed over the years.
Before the widespread adoption of solar technologies, there were initial setbacks and hurdles to overcome. From the first silicon cells developed in the 1950s to modern designs integrating advanced materials, the journey of solar technology showcases innovation informed by both scientific principles and practical applications.
Fundamentals Explained
To truly appreciate solar cell diagrams, one must grasp the underpinning concepts that fuel their operation. At the heart of solar energy systems lies the photovoltaic effect, which converts solar energy into electrical energy.
- Semiconductors: These materials are the backbone of solar cells, typically composed of silicon, which allow the flow of electricity when exposed to light.
- P-N Junction: This crucial interface in solar cells creates an electric field that directs charge carriers, essentially converting photons into usable electric current.
- Modules and Arrays: Understanding how individual solar cells come together in series and parallel configurations to create larger modules is key in grasping solar system designs.
As we explore key terminology, terms like "efficiency," referring to the percentage of sunlight converted into electricity, and "renewable energy," which encompasses energy sources that replenish naturally, are fundamental in cementing this knowledge.
Practical Applications and Examples
Solar energy isn't just theoretical; it manifests in various forms in our daily lives. Think about residential solar panels on rooftops, which utilize solar cell technology to reduce energy bills by tapping into a clean energy source.
Here are examples of practical applications:
- Solar Farms: Large areas filled with solar panels generating power for grid systems.
- Charging Stations: Facilities powered by solar energy for electric vehicles, promoting a sustainable transportation future.
- Wearable Technologies: Devices like watches that use miniature solar cells for charging assist.
Moreover, hands-on projects, such as building a simple solar-powered device, can greatly enhance understanding. An example project could involve creating a small solar oven using a solar panel, tin foil, and oven-safe materials.
Advanced Topics and Latest Trends
As technology rapidly evolves, so does the domain of solar energy. Among the recent advancements are:
- Bifacial Solar Panels: These panels generate electricity from both sides, boosting energy capture.
- Floating Solar Farms: Utilizing water bodies for solar installations alleviates land use concerns.
- Building-Integrated Photovoltaics (BIPV): Solar cells embedded within building materials, like windows or rooftops, present a seamless integration into urban landscapes.
The future of solar energy looks promising, with innovations like perovskite solar cells ramping up efficiency even further. As these technologies continue to advance, the overall growth trajectory of clean energy solutions remains immensely positive.
Tips and Resources for Further Learning
For those wanting to dig deeper into the field of solar energy, various resources could prove helpful:
- Books: "Solar Energy: The Physics and Engineering of Photovoltaic Conversion, Technologies and Systems" is a comprehensive text for understanding both concepts and applications.
- Online Courses: Websites like Coursera or edX often host courses related to solar energy and technology.
- Forums: Engaging in discussions on platforms like Reddit or Facebook can provide shared insights among enthusiasts and professionals in the industry.
By navigating through the significant elements of solar cell diagrams and their practical implications, one develops not just knowledge but a richer understanding of how we can harness the sun's boundless energy. As we step towards a future powered by renewables, the mastery of such fundamental concepts becomes a stepping stone in this transformational journey.
Foreword to Solar Energy
In a world where energy demands are skyrocketing and environmental concerns loom large, solar energy has emerged as a front-runner in the race towards sustainable power solutions. Understanding solar energy is not just about knowing how to harness sunlight; it’s about grasping the pivotal role it plays in combating climate change, maximizing energy independence, and steering the planet toward a greener future.
The Importance of Renewable Energy
Renewable energy sources, particularly solar, offer a myriad of advantages that cannot be ignored. For one, solar energy is abundant. The sun emits enough energy in one hour to power the globe for a year. This means, theoretically, we could tap into this reservoir perpetually without depleting it.
- Environmental Impact: Unlike fossil fuels, which release carbon dioxide and other pollutants when burned, solar energy production releases minimal or no emissions. This clean energy alternative helps mitigate air pollution, fostering a healthier environment.
- Economic Benefits: Investing in solar technology not only reduces electricity costs for consumers but also stimulates job creation in engineering, manufacturing, and installation sectors. In this age of tech acceleration, careers in solar energy are expected to burgeon.
- Energy Security: By harnessing solar energy locally, countries can reduce reliance on imported fuels. This enhances national security and stabilizes energy prices.
In light of these points, it becomes evident that exploring the intricacies of solar technology, including the solar cell diagram, is crucial. This diagram serves as a fundamental guide in understanding how solar systems function, paving the way for a comprehensive grasp of renewable energy.
Overview of Solar Technology
Solar technology encompasses a range of methods used to convert sunlight into usable energy forms. The principle foundation lies in photovoltaic systems, which transform light directly into electricity through solar cells.
These cells possess a unique design involving two layers of silicon—a common semiconductor material. Here’s a quick breakdown of how it all hangs together:
- Solar Cells: At the core of solar technology, solar cells capture sunlight and convert it to electrical energy.
- Inverters: Once solar cells generate electricity, inverters convert this direct current (DC) into alternating current (AC) suitable for everyday use in homes and businesses.
- Battery Storage: To allow utilization of solar energy even when the sun isn’t shining, battery systems store excess energy for later use.
What is a Solar Cell?
Understanding what a solar cell is, forms a cornerstone for grasping the entire concept of solar energy harnessing. Solar cells, also referred to as photovoltaic cells, are the fundamental building blocks of solar panels, the gadgets that help us convert the sun’s energy into usable electricity. This section will delve into what a solar cell does, how it functions, and why it's meaningful to anyone interested in renewable energy technology.
Definition and Functionality
In very simple terms, a solar cell converts sunlight directly into electricity through the photovoltaic effect, a process that creates electrical energy from light. At its core, a solar cell consists of semiconductor materials, which are typically silicon-based, that facilitate the flow of electrons when exposed to sunlight. This means that the more sunlight the solar cell absorbs, the more electricity it can generate.

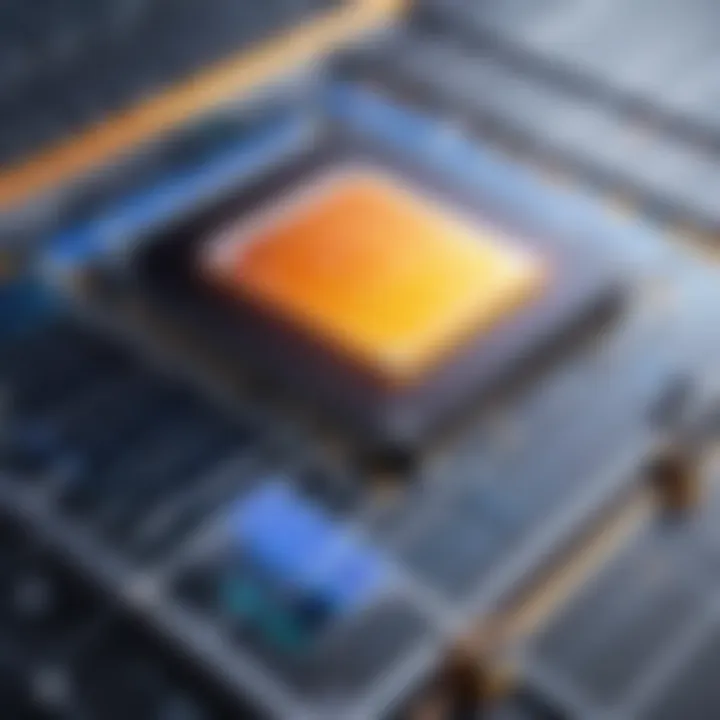
One important point to note about solar cells is how they operate in a eco-friendly manner. Instead of burning fossil fuels or relying on potentially harmful energy sources, solar cells leverage an abundant resource—the sun—making them a sustainable option in our quest for clean energy.
Looking deeper, a solar cell’s functionality can be seen as a series of steps:
- Sunlight Absorption: Photons from sunlight strike the solar cell.
- Electron Excitation: The photons excite electrons in the semiconductor.
- Electricity Generation: Excited electrons move, generating a flow of electric current.
- Current Collection: Conductors in the cell capture and transmit this current for use.
Types of Solar Cells
To better appreciate the topic of solar cells, it’s essential to explore the different types available today. The efficiency, cost, and application of solar technology heavily rely on the type of solar cell being employed.
Monocrystalline
Monocrystalline solar cells stand out for their remarkable efficiency and aesthetic appeal. Made from a single continuous crystal structure, they often feature a uniform dark color, giving them a sleek look. One of the key characteristics that make monocrystalline popular is their higher efficiency rate compared to other types—usually around 15-22%.
This efficient performance comes at a price, as they tend to be more costly to produce, which means that the initial investment is higher. However, many people find their longevity and efficiency make them worth the cost. They have a unique feature of performing better in low-light conditions compared to other cell types.
In summary, monocrystalline cells represent a beneficial choice for those looking for space savings and efficiency, despite their steep price tag.
Polycrystalline
Polycrystalline solar cells are hailed for their cost-effectiveness and solid performance. They are composed of multiple silicon crystals melted together, which results in a speckled blue appearance. One of the main advantages of polycrystalline cells is that they are less expensive to manufacture, leading to a lower cost per watt for consumers.
However, this cost efficiency is accompanied by a slight dip in efficiency, typically ranging from 13-16%. While they may not achieve the same efficiency as monocrystalline cells, their affordability makes them an attractive option for large scale installations, especially in areas where space is abundant.
The unique feature of polycrystalline is their easier production process, making them a popular choice among manufacturers and consumers who are mindful of their budget.
Thin-Film
Thin-film solar cells represent a substantial shift in technology, offering flexibility and a lightweight option when compared to their crystalline counterparts. These cells are made from layers of photovoltaic material only a few micrometers thick, leading to a less rigid structure. Their primary advantage is versatility in installation—thin-film can be applied to surfaces that crystalline cells cannot, such as curved roofs or even some fabrics.
However, the efficiency of thin-film cells typically hovers around 10-12%, making them less efficient than monocrystalline and polycrystalline options. This lower efficiency might render them less desirable for isolated use; however, they shine in niche areas where space and weight concerns come into play.
In summary, thin-film solar cells are beneficial for unique installations, as long as one is not too focused on high efficiency.
The choice of solar cell depends greatly on factors such as application, budget, and space availability. Understanding the differences will help make an informed decision.
Components of a Solar Cell Diagram
Understanding the components of a solar cell diagram is crucial for grasping how solar energy technologies operate. Each part plays a significant role in the conversion of sunlight into usable electricity. By breaking down these components, we can appreciate their individual contributions to the overall functionality of solar cells. This knowledge is not only valuable for students and IT professionals but essential for anyone interested in the evolving field of renewable energy.
Photovoltaic Material
At the heart of every solar cell is the photovoltaic material, which serves as the medium that converts solar energy into electrical energy. The most commonly used materials are silicon-based, but there are alternatives like cadmium telluride and copper indium gallium selenide. Each material affects the cell’s efficiency, durability, and cost.
Understanding the characteristics of these materials is key. For instance, monocrystalline silicon cells are known for their high efficiency and longevity. However, they can come at a premium price. In contrast, polycrystalline silicon tends to be less efficient but is more affordable, making it a popular choice for many residential installations.
- Silicon Types:
- Monocrystalline: Highest efficiency, longetivity, higher cost.
- Polycrystalline: Good efficiency, lower cost.
- Thin-film: Flexible, lower efficiency, lighter weight.
Choosing the right photovoltaic material depends on a balance of budget, efficiency needs, and space constraints in solar installations.
N-Type and P-Type Semiconductors
The functionality of solar cells hinges largely on the N-type and P-type semiconductors. These doped materials create an electric field within the solar cell, which is pivotal for generating electricity. N-type semiconductors are infused with elements like phosphorus that add extra electrons, while P-type semiconductors use materials like boron that create holes, or positive charge carriers.
This doping process fundamentally alters the electrical properties of silicon, enabling the creation of a junction where the N and P layers meet. When sunlight strikes the solar cell, it energizes the electrons, causing them to move from the N-type side to the P-type side. This movement generates a flow of electricity. Understanding this electron flow is essential to grasping how solar cells work effectively. Key points include:
- Electron Movement: N-side to P-side creates current.
- Charge Separation: The junction facilitates the collection of electric charge.
"In simple terms, the interplay between N-type and P-type layers forms the engine of the solar cell."
Conductors and Circuit Elements
Finally, conductors and circuit elements are essential for harnessing the electrical current produced by solar cells. The conductors, typically made of metals such as silver or aluminum, allow electrons to flow from the solar cell to the inverter and, eventually, to your home. The efficiency of the conductors can greatly impact overall system performance.
Circuit elements, like diodes and inverters, also play a vital role. Diodes prevent backflow of electricity, ensuring that the energy produced is not wasted. Inverters convert the direct current (DC) generated by the solar cell into alternating current (AC), which is what most household appliances use.
- Key components include:
- Conductors: Facilitate electron flow.
- Diodes: Control backflow; improve safety.
- Inverters: Convert DC to AC; essential for home use.
How Solar Cells Generate Electricity
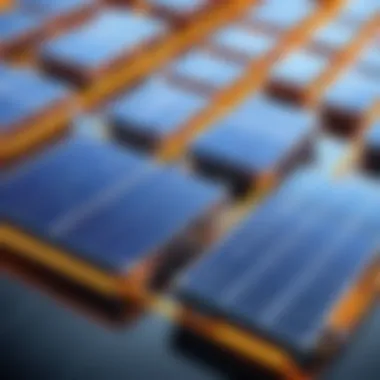
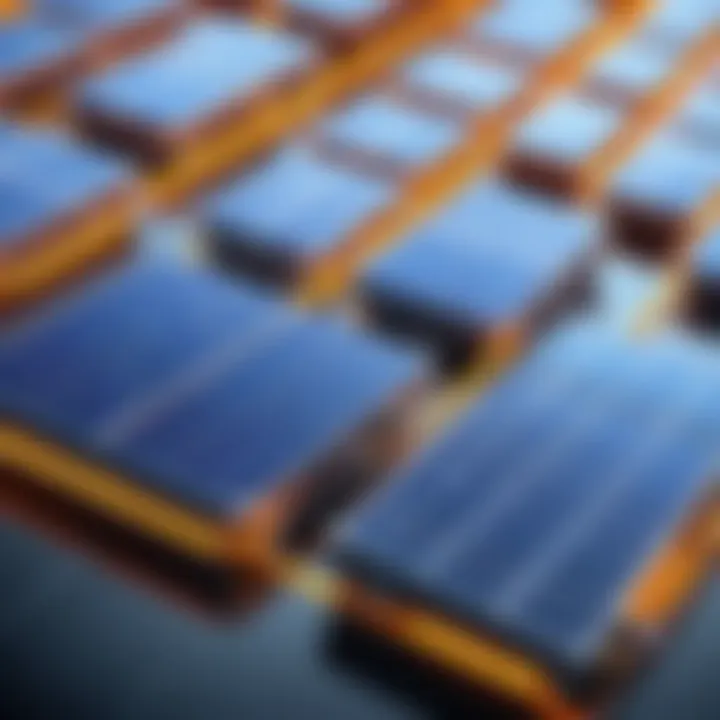
Understanding how solar cells generate electricity is crucial for grasping the overall function and effectiveness of solar energy technologies. This process not only allows us to tap into renewable energy sources but also emphasizes the significance of solar power in combating climate change and promoting sustainability. The energy transformation that occurs within solar cells represents a turning point in how we harness nature’s resources, ultimately leading to reduced reliance on fossil fuels.
The Photovoltaic Effect Explained
The core concept behind how solar cells work is the photovoltaic effect. This phenomenon occurs when certain materials, known as semiconductors, absorb sunlight and convert it into electrical energy. Essentially, sunlight strikes the semiconductor material, typically silicon, and energizes its electrons. When electrons gain sufficient energy, they are kicked loose from their bonds, enabling them to flow freely.
Indeed, this free movement of electrons is where the magic begins. The resulting flow creates an electric current, which can be harnessed for various applications. What’s remarkable about the photovoltaic effect is not just its simplicity but its effectiveness in producing clean, renewable energy without immediate exhaust or pollution.
Conversion of Solar Energy into Electrical Energy
The journey from sunlight to usable electrical energy involves several steps. Once light is absorbed and electrons are freed, two key processes come into play: Charge Carrier Movement and Electrical Flow.
Charge Carrier Movement
Charge Carrier Movement refers to the journey of the freed electrons as they move through the semiconductor material. When light hits the cell, pairs of electrons and holes (the absence of electrons) are created. The semiconductor structure usually includes both N-type and P-type materials, creating an internal electric field. This electric field essentially pushes the electrons toward the front surface of the cell, while holes move toward the back.
This movement is significant; it’s what generates a voltage difference across the cell, allowing for electricity to flow. A key characteristic of Charge Carrier Movement is its efficiency in facilitating this process. Typically, this mechanism can lead to high efficiency rates in converting solar energy to electrical energy. However, factors such as temperature and material quality can influence efficiency, showcasing both the advantages and challenges intrinsic to solar cell technology.
Electrical Flow
Once electrons are mobilized, the next crucial phase is Electrical Flow. This refers to the movement of electrons as they travel through the circuit to provide power to an external load, such as a household device or a battery. As the electrons flow, they transfer their energy to the connected devices, allowing them to function.
A distinguishing feature of Electrical Flow in solar cells is its ability to generate direct current (DC). While most of our electric devices use alternating current (AC), an inverter can convert this DC to AC, making it suitable for everyday use. A popular aspect of Electrical Flow is that it allows for decentralized energy generation, meaning that electricity can be produced directly at the point of use, reducing transmission losses.
However, challenges exist here too. Variability in solar intensity can lead to fluctuations in energy output. This inconsistency poses a challenge for consistent power supply but can be mitigated with energy storage solutions.
"Solar energy is one of the cleanest forms of energy available to us, making its efficient generation vital for sustainable development."
To summarize, the transformation of solar energy into electrical energy through the photovoltaic effect is a complex, yet fascinating process. Understanding Charge Carrier Movement and Electrical Flow gives us insight into the practical and efficient mechanisms solar technology employs to harness renewable resources amidst growing ecological challenges.
Analyzing a Solar Cell Diagram
Understanding a solar cell diagram is essential for grasping how photovoltaic systems operate. Being able to analyze these diagrams effectively reveals the intricacies of solar technology. By looking closely at each component, we can appreciate how they work together to convert sunlight into usable energy.
Analyzing diagrams not only aids in understanding but also enhances troubleshooting skills when issues arise. For technicians and engineers, being adept at reading these diagrams is crucial, as it can be the difference between a smooth installation and costly mistakes.
Key Features to Identify
When examining a solar cell diagram, several key features stand out, each playing a vital role in the functionality of the entire system:
- Photovoltaic Cells: These are the heart of the solar panel. Understanding how they are positioned and interconnected provides insight into efficiency and energy output.
- Conductive Pathways: These indicate how electricity flows through the system. Recognizing the pathways helps in assessing potential bottlenecks or losses in energy conversion.
- Junction Boxes: Often overlooked, these boxes are pivotal for electrical connections and safety. Being aware of their location and function can prevent faults in the system.
- Inverters: Converting direct current (DC) from solar cells into alternating current (AC) is essential for compatibility with home appliances. Identifying inverters on the diagram ensures proper integration into the electrical system.
"A clear understanding of these features empowers users to optimize their solar power systems, making them more efficient and reliable."
Identifying these components not only familiarizes one with the technical aspects but also highlights the importance of each part in the energy generation process.
Standard Symbolography
Every field has its lingo, and solar energy is no different. Within solar cell diagrams, standardized symbols are used to represent various features. Knowing these symbols can significantly enhance comprehension.
- Symbol for Photovoltaic Cells: Typically represented by a rectangle with a wavy line, illustrating the light absorption mechanism.
- Symbol for Electrical Connections: Often shown as circles or dots, these indicate where wires will connect, simplifying the planning for installations.
- Inverter Symbol: It usually appears as a rectangle with arrows, denoting the direction of current flow and its transformation from DC to AC.
- Load Symbol: This can be depicted with a zigzag line, showing where the power is meant to go, be it to appliances or back to the grid.
Familiarity with these symbols can lessen the learning curve and streamline discussions with other professionals in the renewable field.
Each component has its unique representation, which helps avoid confusion when multiple systems are being analyzed or designed. The use of a standardized symbology ensures that everyone is "on the same page", facilitating smoother communication in this specialized area.
By focusing on the features and symbology presented in solar cell diagrams, both students and professionals can enhance their understanding and application of solar technology.
Real-World Applications of Solar Cells
Exploring the real-world applications of solar cells provides critical insight into their growing relevance in today’s energy landscape. The usefulness of solar technology extends far beyond the academic realm, impacting daily life, industry standards, and even global policies aimed at sustainability. By examining various applications, we better appreciate how solar cells contribute directly to overcoming energy challenges and addressing environmental concerns.
Residential Solar Panels
Residential solar panels represent one of the most recognizable applications of solar technology. Homeowners are increasingly turning to these panels as a way to reduce their electricity bills while actively contributing to environmental conservation. The installation of solar panels not only allows families to harness free energy from the sun but also provides autonomy from traditional energy providers. Here are a few key benefits of residential solar panels:
- Lower energy costs: By generating electricity locally, homeowners can significantly cut their monthly energy bills. Many even achieve net-zero energy consumption, leading to potential credits from energy providers.
- Increased home value: Properties equipped with solar energy systems often see an uptick in market value. Prospective buyers appreciate the long-term savings associated with solar installations.
- Environmental impact: Reducing reliance on fossil fuels lowers carbon footprints, leaving a cleaner world for future generations.
However, while there are many upsides, initial installation costs must be carefully considered. Homeowners should research local incentives that can substantially lessen the financial burden. The technological advances that have pushed down the costs of solar equipment are promising, inspiring further household adoption.
Commercial Solar Installations
The commercial sector is also witnessing a surge in solar adoption, with businesses recognizing the value in integrating solar energy into their operations. Large-scale installations on rooftops or in expansive fields allow companies not only to benefit economically but also to enhance their reputations as environmentally conscious organizations. Here are several essential points highlighting commercial solar applications:
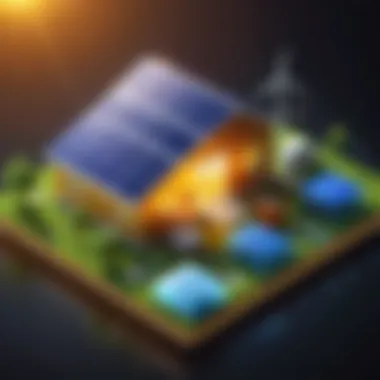
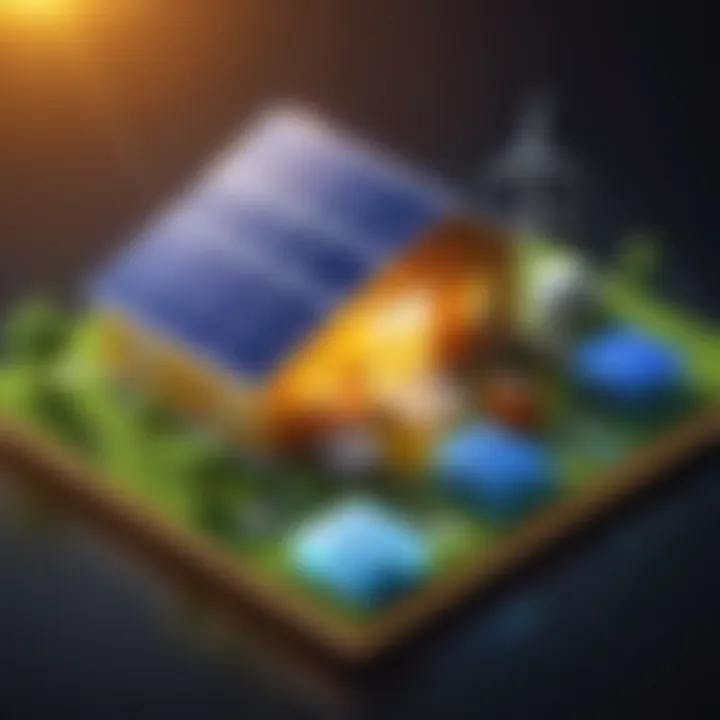
- Cost reductions: Businesses can slash operational costs as solar installations offset a significant portion of electricity expenditures.
- Corporate social responsibility: Going solar is often seen as a commitment to sustainability, improving public image. Companies can market this green initiative to attract eco-conscious consumers.
- Scalability: The flexibility of commercial installations means that businesses can often expand their systems, accommodating growing energy needs without drastic changes.
Nonetheless, businesses must navigate regulatory and logistical challenges. The varying governmental policies on solar energy incentives require careful consideration to maximize benefits. Conducting thorough assessments helps in selecting the right type and size of installation.
Off-Grid Solutions
Off-grid solutions serve as a beacon of hope for those in remote areas lacking access to traditional electricity grids. Solar energy systems designed for off-grid usage allow communities and individuals to break free from dependency on centralized power sources. This application is increasingly vital in various contexts:
- Rural electrification: Many rural communities lack infrastructure for conventional power supply; solar installations provide a sustainable and adaptable alternative.
- Disaster relief: In areas affected by natural disasters, solar-powered systems can quickly restore electricity when traditional sources are down.
- Portable solar generators: These compact systems cater to camping enthusiasts, providing power for small devices without the hassle of bulky equipment.
However, validations for battery storage systems and management considerations are critical since storage capacity directly affects the performance of off-grid setups. Finding reliable technology plays a substantial role in success.
By investing in solar power, society isn't merely chasing economic gains but is also investing in the planet's health—an initiative every generation should endorse.
The integration of solar cells into residential, commercial, and off-grid frameworks illustrates their versatility and importance. These applications continue to evolve with technological advancements, setting a solid foundation for a sustainable future.
Challenges in Solar Energy Utilization
Solar energy, despite its rapid growth and potential, isn't free from its share of hurdles. As the world pivots towards renewable sources, understanding the barriers that affect solar energy utilization becomes essential. This section will delve into the critical challenges related to efficiency limitations and material costs, addressing both the implications for the industry and considerations for future advancements.
Efficiency Limitations
One primary obstacle in the widespread use of solar cells is their efficiency. While technology has advanced significantly over the past few decades, many commercial solar modules still hover between 15% and 20% efficiency. This metric tracks how much sunlight can be converted into usable electricity—a rather disheartening figure when considering the abundant solar energy available daily.
To put it bluntly, not all sunlight ends up doing work. The materials used in solar cells play a role in these limitations. For example, while monocrystalline panels perform slightly better in terms of efficiency, they can be cost-prohibitive for many consumers and small businesses. Other factors that influence efficiency include:
- Temperature Sensitivity: Solar panels tend to lose efficiency as temperatures rise. This effect can be crucial in warm climates.
- Angle of Incidence: The angle at which sunlight hits the solar cell significantly affects output, leading to variability in energy production throughout the day.
- Dust and Debris: Accumulation on panels can reduce output, especially in arid environments. When you've got a layer of dirt, it's akin to throwing shade on your own investment.
Overall, these efficiency bottlenecks pose both engineering and practical challenges, beckoning innovators to devise solutions that enhance performance while lowering costs.
Material Costs and Sustainability
The financial aspects surrounding the production of solar panels are another determining factor in solar energy's adoption. The raw materials, like silicon, can be expensive to source and process. The sustainable practices in extracting these materials are equally critical; when sourcing creates more environmental damage than what it aims to offset, the entire purpose feels undermined.
Moreover, the question of lifecycle sustainability comes into play. As the industry ramps up production, disposing of outdated or broken panels presents environmental challenges. Not all solar cells are designed to be recycled effectively, and as they reach the end of their operational life, the waste generated can be significant.
Considerations worth noting include:
- Material Sourcing: Are we extracting precious resources in a way that can be sustained? Mining practices need scrutiny.
- Recycling Methods: How efficient are current technologies in reclaiming materials from old panels? Smarter methods can turn garbage into gold.
- Future Innovations: With the rise of technologies like perovskite solar cells, there's potential to lower costs and minimize environmental impacts.
"To achieve long-term sustainability in solar energy, we must embrace innovation, not build barriers."
Understanding these challenges helps identify key areas for future research and development, propelling the adjustment needed for more effective and responsible solar energy use.
Future Trends in Solar Technology
The landscape of solar technology is not static; it’s evolving faster than a jack rabbit on a hot tin roof. Understanding future trends in this arena is crucial for anyone involved in renewable energy, as they highlight what’s on the horizon. As concerns over climate change become more pressing, innovations in solar energy technology promise to enhance efficiency, accessibility, and sustainability for the coming generations.
Advancements in Materials
Recent developments in materials used to manufacture solar cells are stirring up a lot of excitement among researchers and industry professionals. Take perovskite solar cells, for instance. These materials are named after the mineral Perovskite, which has a unique crystal structure. Their ability to absorb light and convert it into electricity makes them a game changer. Interestingly, they can be produced through more affordable means compared to traditional silicon cells, cutting down on costs significantly while improving efficiency rates.
For example, while traditional silicon solar cells hover around an efficiency rate of 20-22%, perovskite advancements have already achieved up to 25% in lab settings and show potential for even more. Moreover, their lightweight and flexible characteristics allow for varied applications, from building-integrated photovoltaics to lightweight panels for vehicles.
But material advancements don’t stop here. There is ongoing research into organic photovoltaic materials that promise to be light, flexible, and potentially even biocompatible. This might lead to solar cells that can be seamlessly integrated into fabric or other materials. In other words, your coat might one day charge your devices on the go, blurring the lines between fashion and technology.
Integration with Smart Grids
The relationship between solar technology and smart grids is becoming a topic of great significance, akin to peanut butter meeting jelly. Smart grids use digital technology to improve the reliability and efficiency of electricity services. When integrated with solar technology, they create a symbiotic relationship that maximizes the potential of both.
As solar energy generation typically peaks during daylight, smart grids help in managing that energy efficiently. They allow for real-time monitoring and data flow, which means that energy can be distributed where and when it’s needed most. For instance, during high demand periods in urban centers, excess energy generated by nearby solar farms can be diverted to those areas without a hitch.
Additionally, with the rise of electric vehicles, smart grids can optimize the charging stations, allowing them to draw solar energy during peak generation times and distributing it when needed. This seamless integration not only promotes energy savings but is also a win for sustainability efforts.
In embracing these future trends, individuals and industries can embark on a journey towards a cleaner, more efficient energy landscape. Solar technology is undoubtedly paving the way for a brighter, more sustainable future.
"The integration of solar cells with smart grid technology is like putting a cherry on top of the renewable energy sundae. It makes everything sweeter and more efficient."
Finale and Final Thoughts
As we wrap up our journey through the complexity of solar cell diagrams, it becomes clear that understanding this topic is pivotal for anyone venturing into the dynamics of solar technology. The facets explored in this article, from the intricate components of a solar cell to their real-world implications, are not just theoretical constructs but practical knowledge that can propel innovations in renewable energy.
The importance of solar energy, especially in our current climate, cannot be overstated. It’s not just about harnessing the sun’s rays; it’s about embracing an era where clean, sustainable energy sources become the backbone of our electricity needs. Recognizing the role of various components within a solar cell diagram can lead individuals and industries alike to make educated choices when it comes to adopting solar solutions.
In an age where sustainability is often a buzzword, the practical understanding gleaned from analyzing solar cell diagrams offers tangible benefits:
- Cost Efficiency: Understanding the components helps in choosing the right materials, leading to cost-effective installations.
- Improved Performance: A clear grasp of how energy conversion works can aid in optimizing systems for better yield.
- Sustainability Practices: Knowledge of the ecological impacts associated with solar technology encourages more environmentally responsible choices.
“The future belongs to those who believe in the beauty of their dreams.” - Eleanor Roosevelt. This rings true for the solar industry. Believing in the potential of solar technology is essential for both individuals and society.